Vulnerability and resilience of living marine resources to the Deepwater Horizon oil spill: an overview
- 1College of Marine Science, University of South Florida, Saint Petersburg, FL, United States
- 2Marine Science, Eckerd College, Saint Petersburg, FL, United States
- 3School of Forest, Fisheries, and Geomatics Sciences, University of Florida, Gainesville, FL, United States
- 4Guy Harvey Oceanographic Research Center, Halmos College of Arts and Sciences, Nova Southeastern University, Dania Beach, FL, United States
- 5Harte Research Institute for Gulf of Mexico Studies, Texas A&M University-Corpus Christi, Corpus Christi, TX, United States
- 6Department of Marine Sciences, University of Georgia, Athens, GA, United States
- 7Centre for Research into Ecological and Environmental Modelling, Marine Alliance for Science and Technology Scotland University of Saint Andrews, Saint Andrews, Scotland, United Kingdom
- 8Rosenstiel School of Marine and Atmospheric Science, Department of Ocean Sciences, University of Miami, Coral Gables, FL, United States
- 9Laboratoire de Technologie et Biologie Halieutique, Ecosystem Dynamics and Sustainability (DECOD), French Research Institute for Exploitation of the Sea (IFREMER), INRAE, Institut Agro, Lorient, France
- 10Marine Genomics Laboratory, Department of Life Sciences, Texas A&M University - Corpus Christi, Corpus Christi, TX, United States
The 2010 Deepwater Horizon (DWH) oil well blowout in the Gulf of Mexico (GoM) was the largest and perhaps most consequential accidental marine oil spill in global history. This paper provides an overview of a Research Topic consisting of four additional papers that: (1) assemble time series data for ecosystem components in regions impacted by the spill, and (2) interpret temporal changes related to the vulnerability of species and ecosystems to DWH and the ensuing resilience to perturbation. Time series abundance data for many taxa pre-date DWH, often by decades, thus allowing an assessment of population- and community-level impacts. We divided the north central GoM into four interconnected “eco-types”: the coastal/nearshore, continental shelf, open-ocean pelagic and deep benthic. Key taxa in each eco-type were evaluated for their vulnerability to the circumstances of the DWH spill based on population overlap with oil, susceptibility to oil contamination, and other factors, as well their imputed resilience to population-level impacts, based on life history metrics, ecology and post-spill trajectories. Each taxon was scored as low, medium, or high for 13 vulnerability attributes and 11 resilience attributes to produce overall vulnerability and resilience scores, which themselves were also categorical (i.e., low, medium, or high). The resulting taxon-specific V-R scores provide important guidance on key species to consider and monitor in the event of future spills similar to DWH. Similar analyses may also guide resource allocation to collect baseline data on highly vulnerable taxa or those with low resilience potential in other ecosystems. For some species, even a decade of observation has been insufficient to document recovery given chronic, long-term exposure to DWH oil remaining in all eco-types and because of impacts to the reproductive output of long-lived species. Due to the ongoing threats of deep-water blowouts, continued surveillance of populations affected by DWH is warranted to document long-term recovery or change in system state. The level of population monitoring in the open-ocean and deep benthic eco-types has historically been low and is inconsistent with the continued migration of the oil industry to the ultra-deep (≥1,500 m) where the majority of leasing, exploration, and production now occurs.
Introduction
The oil spill caused by the sinking of the mobile drilling rig Deepwater Horizon (DWH), a well at the Macondo Prospect, was the largest and perhaps most consequential accidental marine oil spill in global history (Lubchenco et al., 2012; McNutt et al., 2012; Powers et al., 2017a). Beginning on April 20, 2010, and uncontrolled for 87 days thereafter, the ~750 million liters of released oil resulted in surface expressions that eventually encompassed over 149,000 km2 (Figure 1). The invisible, subsurface dispersion of oil significantly increased the area impacted (MacDonald et al., 2015; Berenshtein et al., 2020). Because of the origin of the blowout was 1,500 m below the sea surface, transiting oil floated upward through the water column, eventually spreading via surface currents and wind-driven circulation along the continental shelf, and washing ashore on beaches of the northern Gulf of Mexico (GoM) and being entrained in estuaries (Kostka et al., 2011; Powers et al., 2017a; Turner et al., 2019a; Figure 1). Through a still indeterminate combination of natural processes and the use of novel sub-surface dispersant injection (SSDI) into the stream of the flowing oil-gas-water mixture at the broken wellhead, deep submarine “plumes” of small oil droplets formed at several depths, especially at ~1,100 m below the surface (Camilli et al., 2010; Diercks et al., 2010; Murawski et al., 2019). Toxic, low molecular weight components of the oil and gas, especially BTEX (benzene, toluene, ethylbenzene, and xylene) compounds and methane, partitioned into the waters with little reaching the surface (Joye et al., 2011; Ryerson et al., 2012). Through several processes, including oiled marine snow, some of the oil reaching the surface subsequently sank over a wide region of the GoM, impacting benthic communities in a broad swath surrounding the DWH site (Daly et al., 2016; Romero et al., 2017; Schwing et al., 2020, this volume).
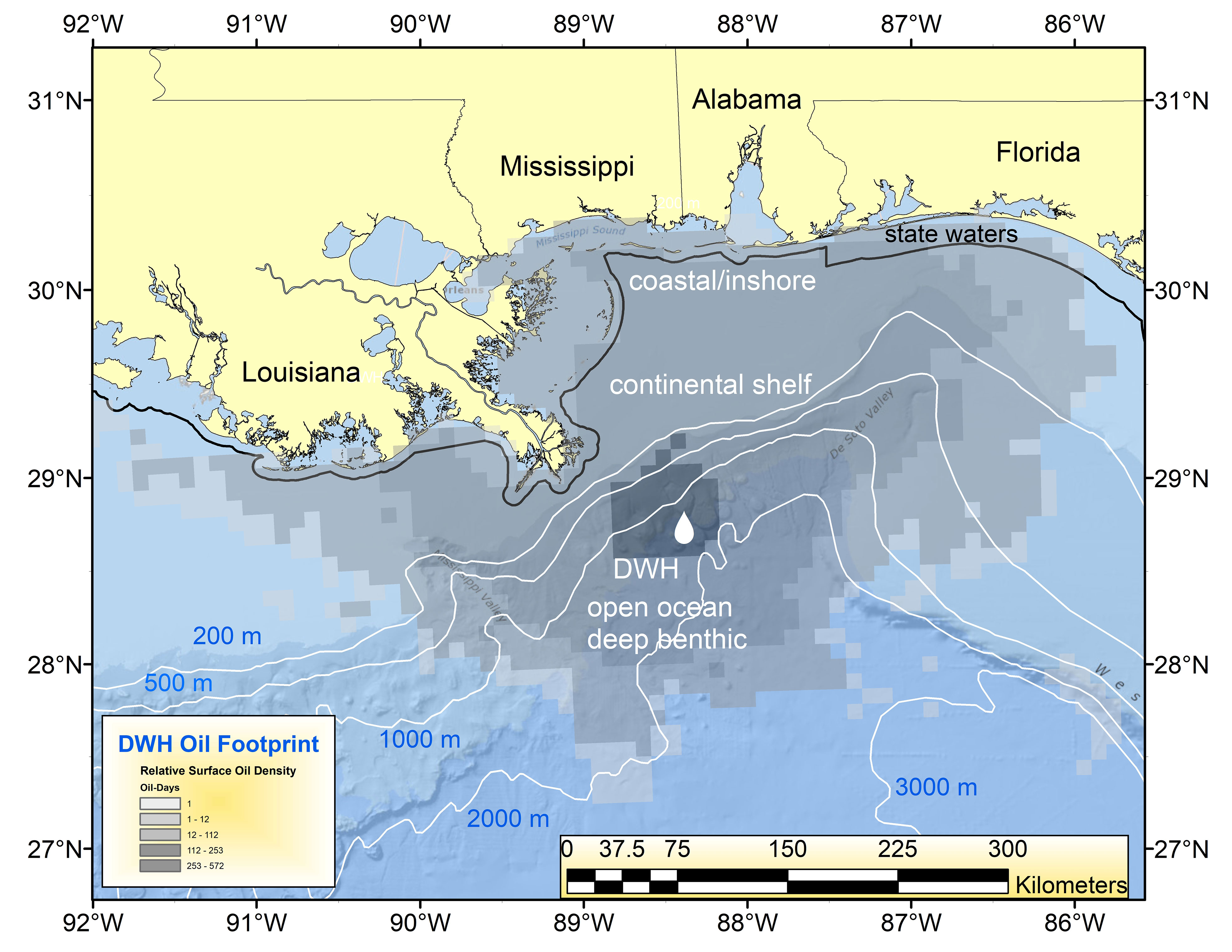
Figure 1 Geographic footprint of surface oil expression (number of “oil days” i.e., days oil was present at each pixel of the oil surface multiplied by the relative oil thickness) for the Deepwater Horizon (DWH) oil spill in 2010 (Murawski et al., 2014). The locations of the spill and four impacted “eco-types” are identified (e.g., coastal/inshore, continental shelf, open ocean pelagic and deep benthic).
A number of oil spill countermeasures were deployed during DWH in efforts to lessen the environmental impacts of the spill (Deepwater Horizon Natural Resource Damage Assessment Trustees, 2016). These included using a total of 6.8 million liters of oil-dispersing chemicals (dispersants) applied both at the wellhead and sprayed by aircraft onto surface oil slicks. Other countermeasures included burning oil at the sea surface, mechanical skimming, booming in coastal areas, opening of diversions from the main channel of the Mississippi River to flood estuaries in an attempt to forestall entry of oil, relocation of nesting animals including sea turtle eggs, and construction of sand berms surrounding sensitive habitats. Apart from the direct environmental effects of oil contamination, these countermeasures also induced a variety of impacts on the ecosystems in which they were deployed (Deepwater Horizon Natural Resource Damage Assessment Trustees, 2016; Powers et al., 2017b). The efficacy of these various countermeasures in lessening environmental impacts is not the subject of this inquiry but has been debated extensively in the literature (e.g., Martínez et al., 2012; Paris et al., 2012; Gros et al., 2017; Powers et al., 2017b; Paris et al., 2018; Murawski et al., 2019; National Academies of Sciences, Engineering, and Medicine, 2020; National Academies of Sciences, Engineering and Medicine, 2022a).
The goal of our five-paper Research Topic published in Frontiers in Marine Science is to provide a decadal synthesis of population-level impacts and evidence of resilience to or coincident with DWH, including the ensuing countermeasures. Attribution of particular environmental impacts to a single source is complicated by a number of factors including the quality and precision of pre-and post-event observations, the underlying variability of association (both spatially and temporally) and the degree of confounding factors affecting the outcome (Ferraroa et al., 2019). Because of the difficulty in evaluating before vs. after and controls vs. impacts (i.e., a BACI design), our approach instead relies on the ‘weight of evidence’ to evaluate probable drivers of observed change in the region affected by DWH. While a number of previous summaries of the population-level ecological effects of DWH have been undertaken (e.g., Fodrie et al., 2014; Beyer et al., 2016; Buskey et al., 2016; Fisher et al., 2016; Murawski et al., 2016; Rabalais and Turner, 2016; Powers et al., 2017a), as well as comparisons made among spills (Barron et al., 2020), it is appropriate to take comprehensive stock of DWH impacts a decade after the spill, particularly as one of the major funding programs supporting this research has now ended active research. The Gulf of Mexico Research Initiative (GoMRI) supported research on the ecosystem impacts of DWH via a $500 million grant from BP and terminated active research in June 2020. There is no doubt, however, that the impacts of DWH on affected ecosystems will continue into the future. For example, Esler et al. (2018) emphasized the impacts on wildlife from the Exxon Valdez spill 28 years subsequent, noting that recovery times of affected animal populations are influenced by species-specific life histories and natural history traits as well as by indirect (food web) effects, chronic exposures (Bodkin et al., 2014), and long-term environmental/climate trends, all of which require the perspectives that only time and intensive research can provide. Esler et al. (2018) also emphasized the dynamic nature of the definition of species and ecosystem recovery and important lessons they gleaned regarding the resilience of species.
Over the course of the previous several years, GoMRI encouraged its researchers, funded in each of seven “Core Areas” (e.g., physical oceanography, oil fate, ecosystem effects, etc.), to synthesize their work emphasizing the following questions:
● What was the state of the science (“baseline”) before Deepwater Horizon?
● What have we learned? (i.e., critical assessment)
● What major gaps in knowledge still exist?
● How can we best apply what we have learned? (What will be the impact – how do we make a difference)?
● Where do we go from here?
To answer these questions relative to Environmental Effects (i.e., the stated goal of GoMRI “Core Area 3”) we assembled a large team of experts from academia, state and federal agencies, and private research entities (i.e., the authorship of the five papers in this Research Topic). Because of the emphasis on scientific baselines, we especially included representatives of state fish and wildlife agencies, all of whom run or sponsor extensive, long-term fishery independent surveys, particularly relevant to the coastal/nearshore eco-type (e.g., O’Connell et al., 2019). As well, the federal government (primarily NOAA Fisheries) conducts parallel surveys of various types mostly focused on continental shelf resources under federal jurisdictions. These long time series were supplemented with mostly post-DWH surveys and directed research on contaminants and health effects conducted by various academic and private research institutions, with funding from GoMRI, the Natural Resource Damage Assessment (NRDA) program, and other sources. The deep ocean water column and benthic eco-types have been historically under-sampled, and our analyses rely mostly upon information collected in the aftermath of DWH, but extending a decade hence. The papers in this Research Topic resulted from two workshops we conducted in 2019:
(1)Assembling the Record of Species and Community Change July 23-25, 2019, St. Petersburg, FL.
(2)Vulnerability and Resilience of Species and Ecosystems to Large-Scale Contamination Events: Lessons from Deepwater Horizon October 9-11, 2019, Washington, DC.
The goals of these workshops were to: (a) identify and obtain data and analyses relevant to interpreting population changes that corresponded in time with the DWH accident, (b) summarize pre- and post-oil spill patterns in the abundance, composition, and dynamics of species within the defined eco-types (see Synthesis Approach section), and (c) construct conceptual models of important species interactions and variables impacting species within each defined eco-type (e.g., Peterson et al., 2003). Additionally, we sought to (d) assess both direct and indirect effects of the DWH on GoM biota, and (e) evaluate resilience and recovery potential of species within each eco-type, and each eco-type as a whole, based on considerations of life history, connectivity within the wider GoM, productivity and exposure potential; as well as evaluate the state of knowledge of rate processes of the key fauna of each eco-type, (f) determine the spatial overlap of species and life stages with the DWH spill, their sensitivity to contaminant exposure, and any other traits influencing species’ vulnerability to the effects of DHW, and (g) provide commentary on the importance of existing monitoring programs and propose additional monitoring given the likely time span to recovery and the potential for future spills. Herein, we describe the overall analytical approach undertaken and define metrics of vulnerability and resilience, or the ability of a population to recover if a disturbance results in abundance over a defined time interval.
Synthesis approach
Because of the three-dimensional and dynamic nature of the DWH spill and the diverse set of affected sub-ecosystems (defined herein as “eco-types”), it is difficult to provide a comprehensive synthesis of effects within the confines of a single research paper. Thousands of species were affected over eco-types ranging from the deep sea to estuaries (Deepwater Horizon Natural Resource Damage Assessment Trustees, 2016). Therefore, we chose to divide our synthesis efforts into four component eco-types comprising the larger north central GoM (Figure 1). These eco-types are: (1) coastal/nearshore, defined as the estuaries to the limits of state territorial jurisdiction of 3 n. miles (except for Florida and Texas which have 9 n. mile state boundaries), (2) continental shelf (from state boundaries to ~200 m water depth), (3) open ocean-water column, and (4) deep benthic (~200 m and deeper). The ecosystem effects of DWH are described in detail in the four eco-type papers comprising this Research Topic (Schwing et al., 2020; Murawski et al., 2021a; Sutton et al., 2022; Patterson et al., 2023). We of course recognize the interconnectedness of the eco-types and the dangers of assuming artificial boundaries among them. This inter-eco-type connectivity is facilitated by the movement of water masses, which distributed DWH oil from a 0.5 m diameter pipe in the deep benthic realm to significantly impact all eco-types (Deepwater Horizon Natural Resource Damage Assessment Trustees, 2016; Berenshtein et al., 2020). As well, many of the component species primarily associated with particular eco-types have life stages that occupy different or multiple eco-types over the spans of their lives. Thus, the eco-types are not considered highly modular (e.g., Grilli et al., 2016) and connectivity is relatively high among as well as within eco-types (e.g., Paris et al., 2020; Sutton et al., 2022).
Membership within the eco-type sub-groups was sought among those actively working on relevant aspects and in control of data resources, including many data series not previously evaluated in the formal published literature for DWH-related effects. This included station-level survey data from state, federal and academic research programs. Thus, the papers in the Research Topic provide new analyses of primary data sources as well as syntheses of previously published information.
Each eco-type sub-group was asked to consider the following target questions in their synthesis.
● For those species or species groups with sampling histories pre-dating DWH, are there measurable differences in abundance and variability pre- and post DWH?
● For those species or species groups measured periodically after DWH, is there evidence of population change (positive or negative)?
● If a species initially declined after DWH, has the population returned to pre-spill or during-spill population abundance levels?
● Can the rates of population decline and/or increase (e.g., instantaneous rates) be estimated?
● If so, are the rates of decline different from the subsequent rates of population increase?
● How do life history correlates (e.g., maximum longevity, larval dispersal, recruitment rate, growth rate, fraction of the population affected by DWH, etc.) relate to population trajectories?
● Is there evidence for hysteresis or, conversely, regime shift in the recovery phase?
● What are the general “take home” messages about environmental impacts and long-term resilience of the GoM with respect to DWH, and lessons for future large oil spills?
The four eco-type syntheses provide details on time series and related data sets considered, and evaluated patterns of change at population and community levels. This review includes, where available, reference to associated contaminant data and other evidence of the effects of direct oil contamination. If applicable, eco-type reviews evaluate the influences of oil spill counter-measures, including effects of the extensive fishery closures in the region (Cockrell et al., 2019; Berenshtein et al., 2020), which, at their maximum extent (229,000 km2), were greater than the visible surface expression of the spill (149,000 km2). The eco-type reviews also consider the limitations of the available data sets (time series length, precision to quantify change, etc.) and describe changes, or lack thereof, in relation to the timing of DWH. In this regard there are a few distinct patterns into which the time series data generally fit: (1) species (or groups) exhibiting a decline in population abundance or change in demography coincident with DWH (with or without subsequent recovery), (2) species (or groups) remaining stable during and after DWH, (3) species (or groups) increasing since DWH, and (4) species (or groups) that initially increased during and just after DWH but have since resumed their “normal” (lower or trending) levels (e.g., as in the case of some populations protected by the fishery closures but relatively unaffected by the spill). Interpretation of the significance of these trends is contingent upon the precision of the underlying surveys which were not, in many cases, developed for the expressed purpose of indexing oil spill impacts over relatively small spatial scales. In these cases, trends in populations are probably more robust than the interpretation of year-to-year changes.
In addition to evaluating population status before, during, and after DWH, each eco-type summary evaluated the vulnerability of the populations assessed to the circumstances of the Deepwater Horizon spill and the resilience potential of each population, in a two-way vulnerability-resilience analysis (see Vulnerability-Resilience Analysis section). Inferences regarding the likely effects of the spill, rates of population change post-spill and progress to population recovery are evaluated.
Vulnerability-resilience analysis
In addition to evaluating population status before, during and after DWH, each eco-type summary evaluated the vulnerability of the populations assessed to the circumstances of the Deepwater Horizon spill and the resilience potential of each population, in a two-way vulnerability-resilience (V-R) analysis. Inferences regarding the likely effects of the spill, rates of population change post-spill, and progress to population recovery were evaluated. While there are many, usually similar, definitions of vulnerability and resilience, herein we define vulnerability as the resultant impacts due to exposure to DWH oil or countermeasures used to mitigate spill effects. Resilience is defined as the capacity required to recover from such effects. From the perspectives of natural resource management, knowing the most vulnerable and least resilient components of a community of plants and animals fulfills several fundamental principles related to understanding the sustainability of those populations in the face of natural and anthropogenic disturbances. More protections are generally afforded to the least resilient (productive) species or groups, and where possible, threat mitigation is used to reduce vulnerability particularly of less resilient components (Carter et al., 2014). There is a long history of published literature and multiple definitions exist regarding resilience of populations (generally thought of as an intrinsic property of populations and ecosystems), and the vulnerability of populations to extrinsic impacting stressors (e.g., Holling, 1973; Gunderson, 2000; Gunderson and Prichard, 2002; Adger, 2006; Adger, 2006; Weißhuhn et al., 2018; Anjos and de Toledo, 2019). Several reviews of definitions have conflated the concepts of vulnerability and resilience (e.g., Carpenter et al., 2001; De Lange et al., 2010a; De Lange et al., 2010b) but it is useful to separate the concepts if we seek to use results from a particular analysis to extrapolate to a different set of circumstances of a stressor (e.g., a spill similar in magnitude to DWH, but at a different place and time) and the resulting affected community of species with (likely) differing life histories.
The separation of components of vulnerability and resilience has precedent in natural resource management fields outside of oil spill effects assessment, including ecosystem-based management (Levin and Lubchenco, 2008), and fisheries management. Operationalizing the assessment of the vulnerability and resilience in fisheries management has a parallel in the so-called productivity-susceptibility analysis (PSA). PSA is a process wherein the operational characteristics of a fishery (e.g., bycatch species and magnitude, size and age selectivity of a fishing gear, etc.) are used to define the susceptibility axis. The productivity potential of a species, defined by population dynamics metrics (i.e., rates of growth, natural mortality, fishing mortality, recruitment) is used to define how productive, and thus how resilient a stock may be if it becomes depleted (Stobutzki et al., 2001; Hobday et al., 2011). PSAs have been implemented globally as requisite data are routinely available for many regulated fisheries, and in particular, an expert-based qualitative approach has most commonly been used to define risk horizons for low productivity species subject to mixed-species fisheries (e.g., elasmobranchs, rockfishes and other long-lived species of limited reproductive capacity; Field et al., 2010).
In the fisheries context, high risk (defined either by high susceptibility or low resilience) may undermine attainment of sustainability goals for the species in question. These goals for fisheries management are a combination of population size targets and/or fishing mortality thresholds (Field et al., 2010; Patrick et al., 2010). Most applications of PSA use a three-level qualitative scoring system (low, medium, high levels of susceptibility and productivity) with scores averaged for each attribute contributing to susceptibility and productivity, among experts participating in the analysis. The average scores by attribute are then summed for each axis independently. The results are plotted in bivariate space on which a risk continuum (zones of equivalent risk for each x,y pair of possible scores) are overplotted as either lines or as gradient shading (low to high risk) representing the response variable (Patrick et al., 2010). However, this semi-quantitative algorithm is controversial (e.g., Hordyk and Carruthers, 2018), due to the general lack of testing of PSA categorization against quantitative models of species population dynamics and because of the assumption that scores on the various criteria are additive. However, the methodology we used to evaluate resilience employed the observed trajectories for biota affected by the DWH spill, which we consider to be more informative of resilience potential than interpreting theoretical population model for the key species.
We co-opted the PSA approach but modified it to pertain specifically to the vulnerability of a species or species group to impacts from the DWH spill, and its resilience to perturbations on scales seen following the spill. Vulnerability (the equivalent term to susceptibility used herein) in these analyses is specifically related to the characteristics of DWH, including the timing, overlap of oil with species and their life stages (e.g., Chakrabarty et al., 2012; Chancellor, 2022), concentrations of oil components above toxic thresholds for the biota, and other factors. In total, 13 attributes were scored categorically to estimate a given taxon’s overall vulnerability to the DWH spill (Table 1). For each attribute, its interpretation (directionality) was evaluated resulting in low, medium or high vulnerability determinations (Table 1). In most cases, the directionality is obvious, for example, with respect to the attribute of duration and frequency of acute/chronic exposure (persistence), it is assumed that longer duration of exposure results in greater vulnerability of a population than if exposure duration is short. We recognize that a number of attributes are not completely independent and thus our approach to synthesizing across attributes for a single vulnerability assessment was designed to be relatively insensitive to the inter-independence of some attribute scores.
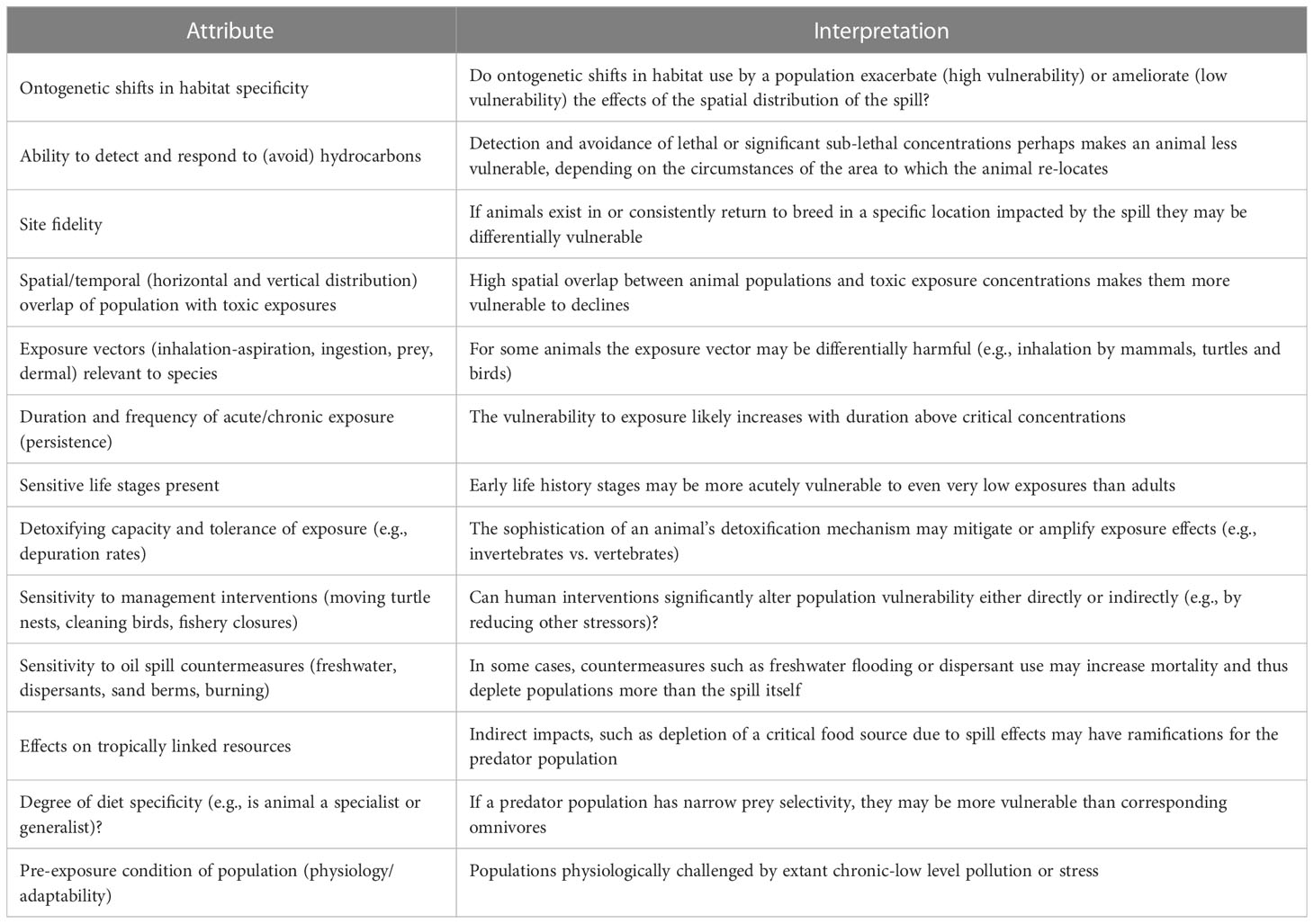
Table 1 Attributes influencing vulnerability of marine life specifically to the circumstances of the Deepwater Horizon oil spill.
Similar to the vulnerability axis, we defined 11 attributes contributing to resilience of species populations (Table 2). The directionality of resilience scores for each attribute are likewise generally obvious. For example, highly fecund species are likely to exhibit higher resilience to perturbations than less fecund ones.
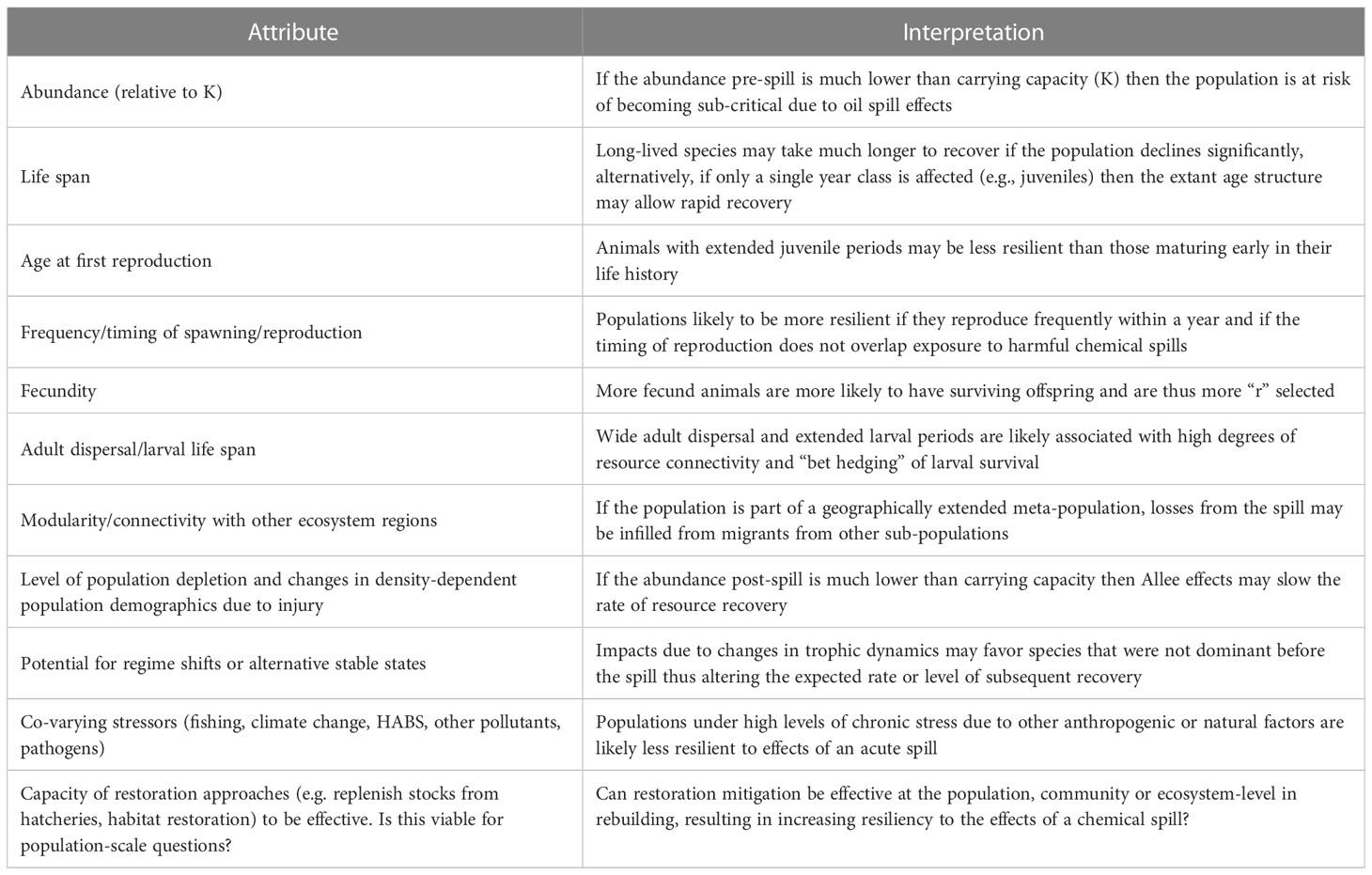
Table 2 Population attributes influencing resilience of species populations to impacts from a chemical spill in the context of other simultaneous stressors.
Each eco-type group conducted their V-R analyses for a series of key species, thusly: (1) each participant in the eco-type group independently evaluated each vulnerability and resilience attribute for every “key” species using the low, medium and high ranking system, (2) an overall score was assigned based on both the plurality of scores among participants and subsequent group discussion, and (3) based on the scores determined for each of the attributes contributing to vulnerability and resilience, a single categorical score was determined for each key species and axis (i.e., V and R). The attribute scores for three of the eco-types and their respective key taxonomic group are evaluated in eco-type synthesis documents (Schwing et al., 2020; Murawski et al., 2021a; Sutton et al., 2022). We report the outcome from such scoring for the continental shelf synthesis from Patterson et al. (2023).
While there is no universal definition for what constitutes a “key” species, factors considered in these evaluations were: economic importance (e.g., recreational and commercial fishes), role as a critical species in food webs (e.g., McCann et al., 2017; Ainsworth et al., 2018; Dornberger et al., 2020; Lewis et al., 2020), protected species status (Deepwater Horizon Natural Resource Damage Assessment Trustees, 2016; Schwacke et al., 2017; Fraser, 2020), degree of endemism (Chakrabarty et al., 2016), or species of particular vulnerability or importance to the eco-type (e.g., Fisher et al., 2016; Sutton et al., 2020; Sutton et al., 2022). Each eco-type group identified 11-32 key species or taxonomic groups for consideration in their V-R analyses based on the assembled expert judgement (Figure 2).
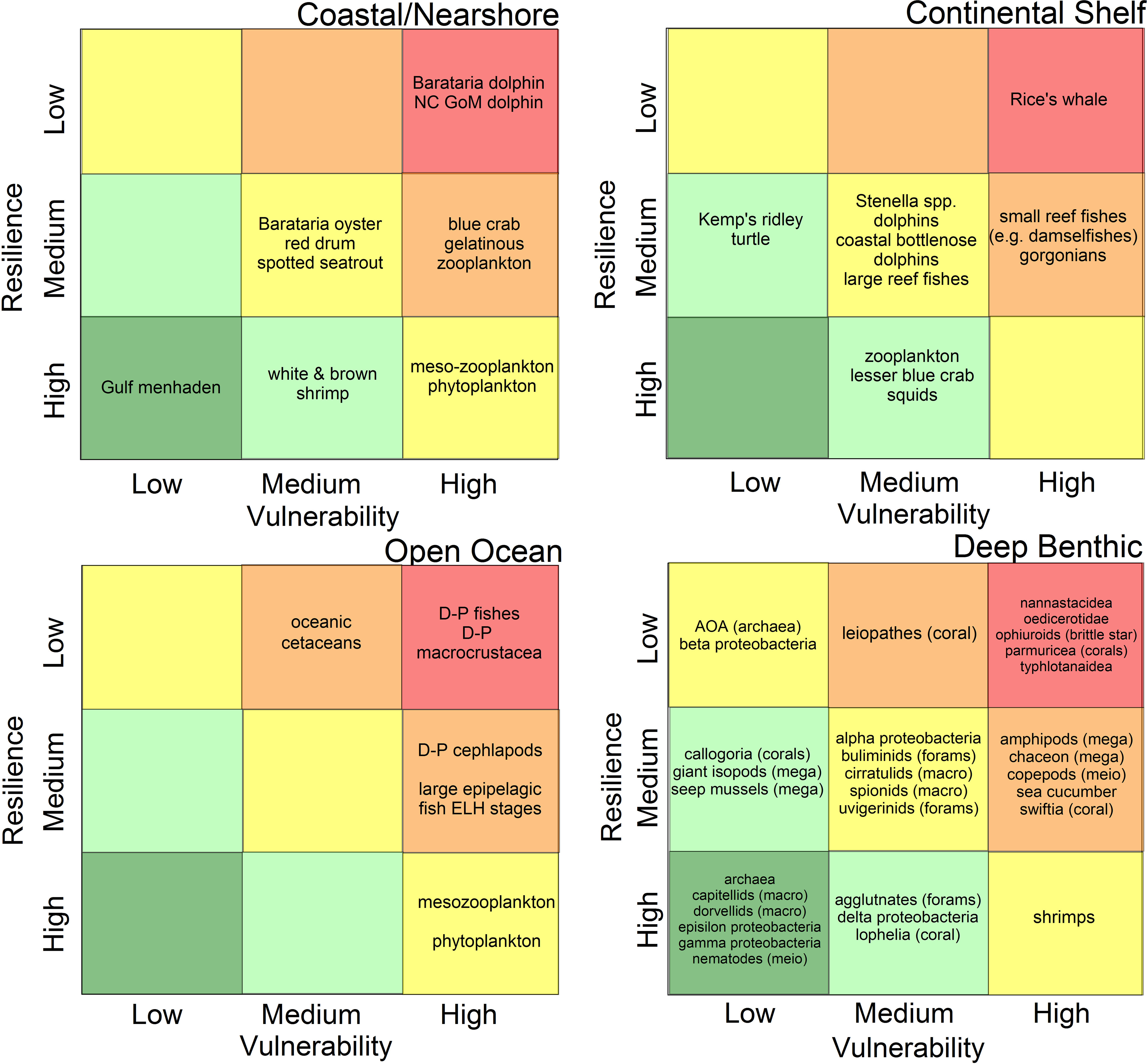
Figure 2 Schematic of the relative vulnerability of species populations to the Deepwater Horizon oil spill and the resilience potential of populations associated with four defined eco-types in the northern Gulf of Mexico. Grid color scheme represents the relative risks of significant impacts from the spill and protracted recovery times (e.g., red = highest risk, dark green = lowest risk). Scientific names of individual species are listed in each of the eco-type documents in the Research Topic. m.m., marine mammals; NC-GoM, North Central Gulf of Mexico; mega, mega-benthos; macro, macro-benthos; meio, meio-benthos; forams, foraminifera; D-P, deep-pelagic (fishes, etc.); ELH, early life history stages.
Within an eco-type, the summary positions along the vulnerability and resilience axes are plotted in one of nine possible cells (Figure 2). The incremental color scheme within the field of the plot gives a broad indication of the relative risk of significant species impacts due to the spill and the relative resilience to perturbation. For example, the deeper red indicates a zone of high vulnerability and low resilience, the combination of which carry relatively high risk.
Discussion
Despite occurring 13 years ago, the magnitude and diversity of effects DWH spill continues to inspire additional analyses and syntheses of the impacts on natural resources of the Gulf of Mexico and provide a rich template for “lessons learned” from that spill. We developed an algorithm to assess and classify the simultaneous vulnerability of marine resources to the specific impacts of the Deepwater Horizon oil spill and the resilience potential of various key species and groups to environmental threats posed by this and similar events. This two-way classification allows for understanding of the idiosyncratic effects of that specific event on species and groups, taking into account the vagaries of life histories (including age span, reproductive potential and population connectivity) affecting the ability of populations affected by spills to recover. A number of recent, somewhat similar, efforts to provide syntheses of recovery potential have focused on various taxonomic groups including marine mammals (Ramírez-León et al., 2023), seabirds (Michael et al., 2022), sea turtles, and various fish/fishery species (Chancellor et al., 2020; Polidoro et al., 2020; Chancellor, 2022; Woodyard et al., 2022). Assessing potential impacts (vulnerability) to previous and potential spills has utilized both spill trajectory modeling (Chancellor et al., 2020; Chancellor, 2022; Ramírez-León et al., 2023) or specific locations from which spills may emanate (e.g., oil and gas platform locations, Michael et al., 2022). These spill scenario studies are particularly useful for planning and response purposes for understanding resources at risk, whereas vulnerability assessments based primarily on life history alone cannot adequately assess impacts associated with the temporal or spatial dynamics of particular spills in relation to distributions of critical life history stages.
The four-panel V-R plots (Figure 2) provide a summary overview of the relative vulnerability and resilience of key species across the span of eco-types affected by the DWH spill. We had thought that this analysis might highlight, at the sub-ecosystem level, those eco-types proving to be more vulnerable and less resilient than the others. In fact, all of the eco-types exhibit a range of species classifications across the breadth of outcomes, with no outstanding trend. While these analyses represent a highly selected sub-set of the literally thousands of species affected by the spill, they do provide important insights into how spill effects transcended the range of eco-types and how idiosyncratic the vulnerability of particular species was to the DWH spill scenario. As well, even within an eco-type, the differential tolerance (and perhaps acclimation and/or adaption resulting from chronic exposure) to oil contamination is not universal among species occupying a similar habitat. Even in animal groups thought to be at particular risk from oil spills (e.g., marine mammals) the variations in vulnerability to DWH effects and the demonstrated resilience within the group was large (e.g., Barataria Bay bottlenose dolphins, Tursiops trunccatus, in the coastal/nearshore vs. mid-depth diving marine mammals in the open ocean). While all of these mammal populations would likely be more similarly impacted had they been consistently exposed to oil, the mosaic of oil concentrations, pre-spill physiological condition, mobility of animals in relation to the presence of oil, and the duration of exposures created a very heterogeneous exposure field. There is some indication that animal size is correlated with resilience (e.g., Schwing et al., 2020; Figure 2), however, this trend was not universal and may reflect the specific circumstances of the DWH spill. For long-lived species (e.g., a decade or more) their life history scheme may be indicative of either high or low resilience, depending on the circumstances of exposure. For example, if a particularly vulnerable (young) year class is heavily impacted but adults are not, repeat breeding by the remaining year classes may mitigate the effects of the partial loss of a single cohort (Gallaway et al., 2016). However, if the entire population (juveniles and adults) is affected, then life history traits may result in long post-spill recovery times (e.g., Schwacke et al., 2017) and hence low population resilience. The determination of resilience potential, based upon attributes described in Table 2 and the trajectories of surveyed species, is less certain for populations whose life histories are poorly known (e.g., most mesopelagic species and those associated with the deep benthos) and thus there are variable degrees of uncertainty in assigning grid location by species and eco-type. Although we did not do so, one could add an additional weighting term or other indication to the risk determination based on the perceived certainty in population dynamics of each key species. Because of the importance of life history data to these evaluations, we deem research to close these gaps critical (see Priorities for Future Research below).
The bivariate V-R plots also have an important assumption of the effects of co-stressors and natural environmental variability implicit within them. These analyses characterize vulnerability to a specific spill (DWH); the observed resiliency is informed by the trajectories of pre-and post-spill population dynamics. In some cases, populations (e.g., blue crab, Callinectes sapidus, in the coastal/nearshore and lesser blue crab, Callinectes similis on the continental shelf) may have been in long-term decline since prior to the DWH spill (O’Connell et al., 2019; Murawski et al., 2020; Patterson et al., 2023), likely due to habitat degradation or fishing pressure. Establishing causal inference regarding a single driver (e.g. DWH) as the agent of change is subject to assumptions of both excludability (i.e., no other drivers of significance) and no interference (i.e., no confounding spatial effects from outside the spill area; Ferraroa et al., 2019). Similarly, the dynamics of recovery of continental shelf populations is confounded by the increased abundance of invasive species (lionfishes, genus Pterois) which may be impeding the recovery of otherwise productive ecosystem components (Lewis et al., 2020; Patterson et al., 2023). Thus, characterizing a species or an eco-type as vulnerable or resilient must be conditioned not only on the oil spill scenario but also the cumulative effects of important co-stressors (e.g., O’Connell et al., 2019). These considerations are important when developing oil spill contingency plans or reacting to an ongoing event. However, few, if any, other stressors operate on the same temporal or spatial scales as DWH, or could be expected to impact as many eco-types as were considered here. Thus, by taking a synthetic view of the eco-types of the GoM, we can begin to better understand the overall population-level impacts of the spill.
These analyses also provide important context when considering environmental trade-offs resulting from the application of optional oil spill countermeasures. For example, French-McCay et al. (2018) considered the use of SSDI at the wellhead as it related to deep ocean and nearshore biota in the format of a Comparative Risk Assessment (CRA). However, as described in the papers in this Research Topic, the trade-off is not a binary choice of sacrificing one eco-type for another. Rather, there are complex and differential species vulnerabilities and resiliencies and, as we note, important connectivities among the eco-types that render this trade-space rather arbitrary and not necessarily protective of important species or species interactions. In our context, for example, the vertically migrating biota of the deep ocean (Sutton et al., 2020; Sutton et al., 2022) link surface and deep-sea communities and provide important ecosystem services including carbon sequestration and food sources for valuable commercial and recreational species (e.g., tunas, billfishes). Thus, such trade-offs may articulate a false dichotomy of “importance” to the environment and the economy based on historic data deficiencies rather than value to the ecosystem, and are no substitute for careful consideration of response options and the totality of their effects, in order to avoid long-lasting and unintended consequences.
Unlike most previous large-scale oil spills (e.g., the IXTOC 1 spill; Soto et al., 2014), the DWH and Exxon Valdez (EVOS) oil spills were accompanied by well-funded, decadal-scale research programs established in their aftermaths. Peterson et al. (2003) conducted a synthesis of long-term environmental effects of the EVOS 14 years after that coastal/nearshore tanker accident. Their review documented important ecosystem-level perspectives in considering the ecotoxicology of marine oil spills. Noting the importance of “…delayed, chronic and indirect effects of petroleum contamination” their insights largely contrasted with previous models that did not consider long-term chronic exposure from sequestered oil on fishes, mammals and birds and further they observed that oil spill countermeasures (“clean-up”) may be more damaging than the spill itself. Our syntheses extend their ecosystem emphases further due to the complexity of the DWH spill and its open-ocean blowout characteristics (Peterson et al., 2012). The EVOS occurred in a coastal fjord, extending southwestward to cover 2,100 km of shoreline and into nearshore regions the Gulf of Alaska (Peterson et al., 2003). DWH was almost the opposite, starting deep offshore and eventually expanding to 149,000 km2 of surface contamination (MacDonald et al., 2015; Berenshtein et al., 2020) and impacting at least four distinct eco-types (Figure 1). The EVOS affected a number of populations with limited breeding ranges, and in particular, birds, mammals, and anadromous and coastal-spawning fishes (e.g., pink salmon, Oncorhynchus gorbuscha, and Pacific herring, Clupea pallasii). Population-level effects of EVOS were widespread and in some cases chronic due to ongoing pollution effects on vulnerable life stages. One important distinction among these two iconic spills is that for EVOS, the spill extended to include a large portion of the home range or spawning grounds of entire populations segments of species. While this is known to have occurred for some species during DWH, (e.g. bottlenose dolphins in the coastal/nearshore) the spill encompassed only parts of population ranges for oceanic and continental shelf and oceanic populations (e.g., red snapper, Lutjanus campechanus, and bluefin tuna, Thunnus thynnus). Even in the coastal/nearshore, many of the GoM’s estuarine populations spawn offshore with larvae transported to the estuaries (Murawski et al., 2021a). Thus, the open ocean nature of the blowout may have mitigated population-level declines (at least for coastal and continental shelf species) because of the spill’s spatial dynamics, migration and passive transport of species and the timing of the spill encompassed only portions of the spawning windows (Chancellor et al., 2020; Paris et al., 2020). Thus, there may have been important portfolio effects (Schindler et al., 2010; Carlson and Satterthwatte, 2011) among sub-populations mitigating species-wide impacts within some eco-types. Paradoxically, however, the significant decline across the board for mesopelagic species following the DWH (Sutton et al., 2022) implies either that there are localized population segments (which is doubtful given their extensive migrations in the vertical and due to horizontal displacement [Milligan and Sutton, 2020]) or that, more likely, that there is low modularity and high connectivity allowing the impacts of the DWH spill to cascade through the micronektonic assemblage, despite the spill covering only a portion of the deep water GoM (Figure 1).
As with EVOS, there remain significant reservoirs of DWH oil in the environment in salt marshes and in the deep benthic realms, which, also like EVOS, will have chronic effects on biota there for perhaps decades to come (Turner et al., 2019a; Schwing et al., 2020). The consequences of the DWH spill for such a wide variety of species and eco-types and potentially over decadal time frames re-enforces the thesis advanced by Peterson et al. (2003) that our perspective for understanding large scale spills has indeed shifted to ecosystem-scale, from a field previously dominated by accounting for effects on individual species.
As Peterson et al. (2003) observed, EVOS countermeasures (in this case including steam-washing of beaches) exacerbated and extended consequential harm to the ecosystem. In the case of DWH, there are analogies not only related to compounding negative environmental effects of counter-measures but these observed effects may portend environmental consequences of large-scale projects intended for ecosystem restoration in the northern GoM. Diversions of water from the main stem of the Mississippi River into the Louisiana marshes freshened the saltmarsh ecosystems in Barataria Bay and Breton Sound (Rabalais and Turner, 2016; Powers et al., 2017b; White et al., 2018). The freshening of the marshes resulted in billions of mortalities of market-sized American oyster, Crassostrea virginica, and other resource and non-resource invertebrates (Powers et al., 2017a). While the proximal cause of dolphin morbidity and mortality was oil exposure (Schwacke et al., 2014), that the population density is higher near the mouth of Barataria Bay, where oil entered, probably exacerbated their average exposure (Murawski et al., 2021a). Ultimately, diverting freshwater into the marshes likely had little effect in forestalling oil entry into these bays where oil accumulated and still exists more than a decade after the spill (Turner et al., 2019a; Murawski et al., 2021a). However, this “experiment” also provides some important insights into potential consequences of long-term habitat restoration activities affecting the coastal/nearshore region off Louisiana (e.g., White et al., 2023). To address the long-term effects of sea level rise and land subsidence in the wetlands, large portions of settlement moneys from the DWH accident are being used to expand and add more water diversions along the Mississippi River to funnel sediments into the marsh and therefore at least partially stabilize land loss (Coastal Protection and Restoration Authority of Louisiana, 2017; Boesch, 2020; Coastal Protection and Restoration Authority of Louisiana, 2023). Furthermore, these diversions will likely release as much or more water than was diverted in 2010 during the DWH spill (White et al., 2018). Notwithstanding the debate regarding the likely effectiveness of these diversions in stemming land loss (Turner et al., 2019b; White et al., 2023), it is reasonable to expect, as observed during the DWH episode, that dolphins, oysters, and other invertebrates and fishes dependent on brackish habitat will be similarly negatively affected (Powers et al., 2017b; White et al., 2018; Murawski et al., 2021a).
The analyses of the vulnerability and resilience of resources to the DWH oil spill fit into a larger conceptual model of how both of these attributes act at various levels of biological organization (organism, population, community and ecosystem, Grosell and Pasparakis, 2021; Halanych et al., 2021; Murawski et al., 2021b), and how they are both influenced by other co-stressors and affected by active and passive resource restoration (Figure 3; Greening et al., 2022). Our review centers around effects at the population and higher levels of organization, but contaminant effects have their origin at the sub-cellular to organismal levels, which are evaluated elsewhere in GoMRI synthesis activities (e.g., Grosell and Pasparakis, 2021; Halanych et al., 2021; Murawski et al., 2021b). As demonstrated in our work and that of others, some of the other co-stressors may have been as or more dominant than DWH population and ecosystem-wide effects (e.g., illustrated by fishery closures effects). Since some of these co-stressors are under human control, they can also be manipulated with the goal of enhancing the rate of resource recovery and potentially affecting restoration targets for “recovery”. In this respect, much of the money being invested from settlements is supporting a long list of habitat change/restoration projects. With sufficient monitoring and analysis (e.g National Academies of Sciences, Engineering, and Medicine, 2022b; Greening et al., 2022), it might be possible to determine if those projects substantially affect resources at the population, community, and ecosystem levels of organization. So-called “compensatory” restoration measures can also strengthen the resilience of populations to future spills and other anthropogenic stressors. With this in mind, there are a number of actions resource managers can take to enhance the resilience of resources in the region to be better able to withstand the impacts of spills on the scale of DWH through more proactive conservation-centric approaches (Wallace et al., 2019), many of which may be more impactful than physical alterations of habitat. These may include expanded use of marine and estuarine protected areas, more conservative fishery management approaches (i.e., to build reproductive capacity), and lowering the thresholds for discharges of other toxic chemicals, among others. Reducing vulnerability and strengthening resilience of coupled human-natural systems within which the chronic and acute effects of oil spills are an ongoing threat requires a more holistic approach to the totality of natural resource management actions (Adger et al., 2005; Adger, 2006; Carter et al., 2014).
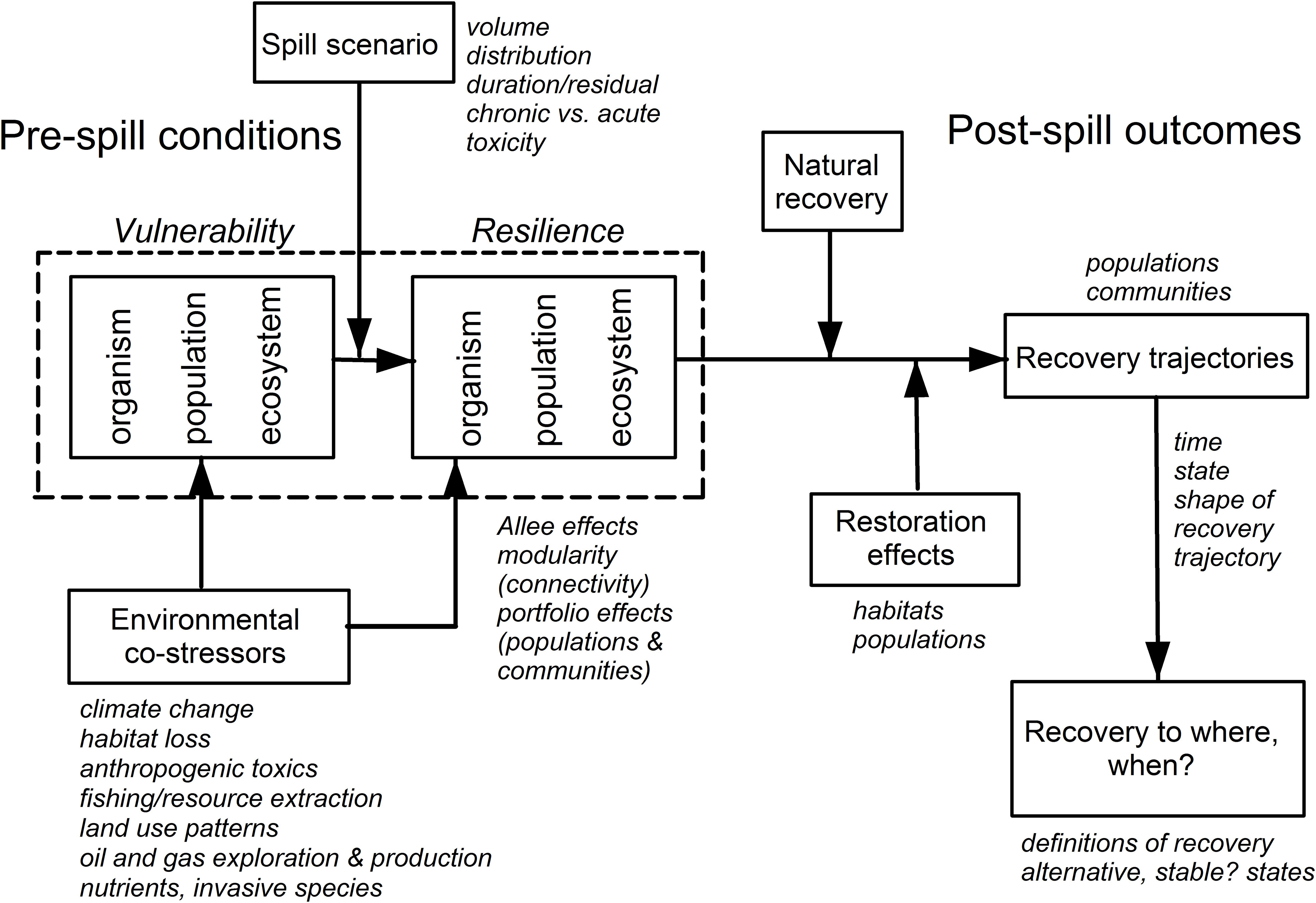
Figure 3 Factors affecting the vulnerability and resilience of organisms, species populations and ecosystems exposed to a Deepwater Horizon-like oil spill. Many co-occurring stressors (e.g., fishing, invasive species) simultaneously affect vulnerability and resilience, as do restoration activities. The shape of the decline and recovery scenarios is not necessarily symmetric, nor are expected recovery levels necessarily equivalent to pre-spill conditions.
Priorities for future research
Despite the preceding decade of research into the causes and consequences of DWH, there remain a number of priority research questions worthy of ongoing study. Some of the most immediate and pressing research needs include:
(1)Monitoring the long-term recovery potential of eco-types (e.g., mesopelagic, deep benthic) and long-lived species to determine if populations and communities eventually return to pre-spill “baselines”, have stabilized at a “new normal”, or followed different trajectories
Prior to the NRDA and then GoMRI-sponsored research associated with DWH there were just a few, sporadic research efforts devoted to indexing the biodiversity and abundance of populations in the open-ocean water column and deep benthos (Rowe and Kennicutt, 2008; Schwing et al., 2020; Murawski et al., 2021a; Sutton et al., 2022; Patterson et al., 2023) and marine mammals and other protected species everywhere. Sampling since DWH in these eco-types has revealed large temporal changes and incomplete return to what were likely pre-spill levels. These regions are a high priority for future targeted research investments because of the continuing expansion of the oil and gas industries into ultra-deep waters of the GoM (Figure 4). Most of the current oil producing and active but non-producing leases exist between 1,000 and 3,000 m water depth (Figure 4). It is imperative that longitudinal studies continue there to document DWH-associated effects and to understand the productivity and sensitivity of these eco-types to exploration, production and to both chronic oil-related pollution and that of acute spills. In this regard, and because of the difficulty in restoring the deep ocean species and habitats affected by DWH, a more prospective approach of “research as restoration” is warranted (Mengerink et al., 2014).
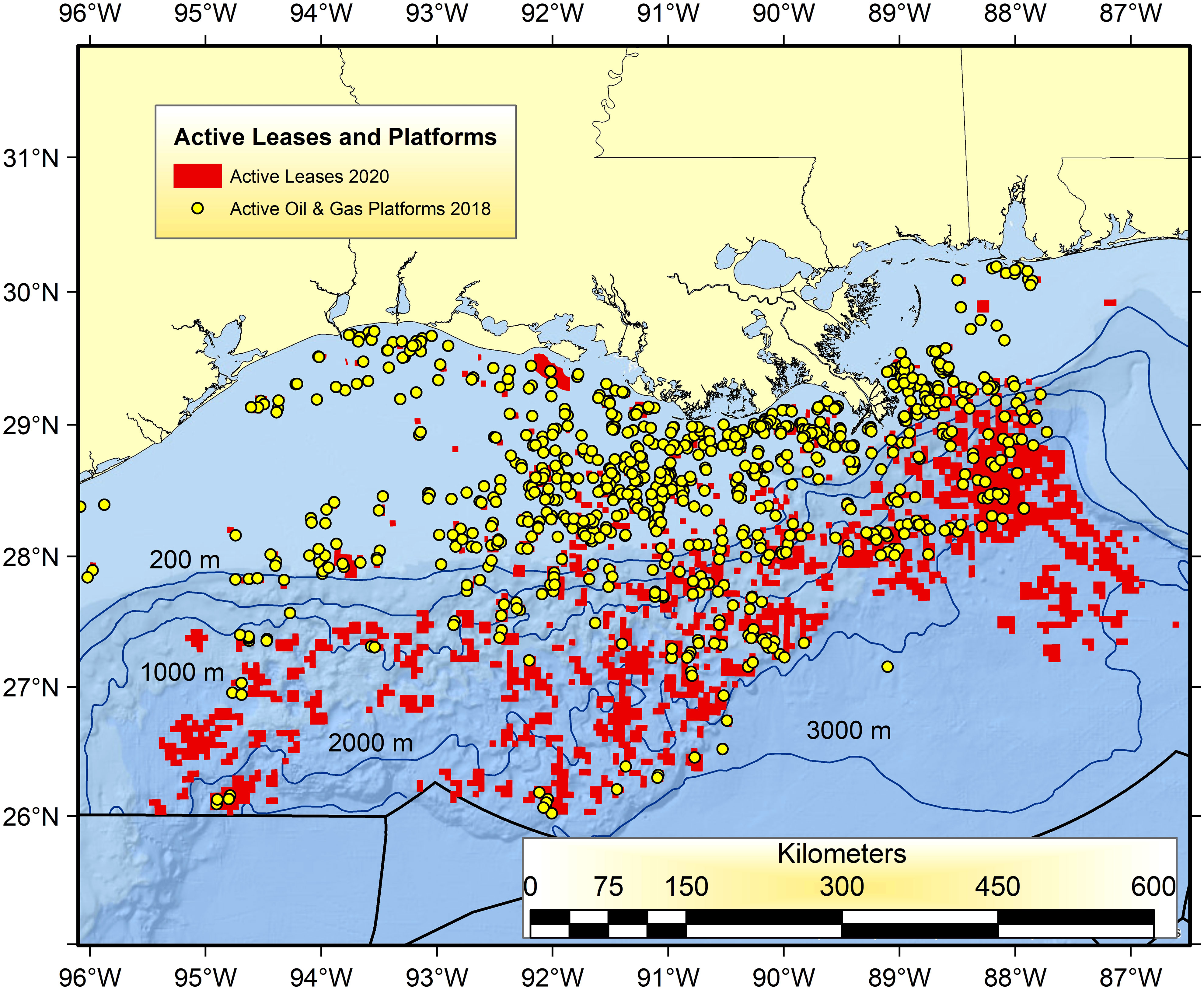
Figure 4 Active oil and gas infrastructure in 2018 (yellow dots) and currently active lease blocks in the Gulf of Mexico (red squares) as of 2020. Lease blocks with no infrastructure indicate locations that are areas already identified by lease holders as having potential for future exploration and production. Data available at: https://www.data.boem.gov/.
(2)Understanding the connectivity/modularity of key living resources and their roles in ecosystem resilience and recovery GoM-wide
As we note above, the relative resilience of many key species to DWH impacts was perhaps due to the connectivity of units within meta-populations with distributions that were broad enough to encompass areas unaffected by the spill. Understanding how larval distributions, adult animal movements and gene flow support resource connectivity networks is an important area for investigation especially given the undoubtedly different circumstances of transport of the next large oil spill in the GoM. A number of elements necessary to fully evaluate the roles of connectiivity contributing to resilience include:
(a) Quantifying the rates of coupling (e.g., meso- to epi-pelagic, bentho-pelagic) among ecosystem components,
(b) Accounting for the advection and fate of coastal resources transported offshore, and vice versa,
(c) Calculating exposure fields and quantifying ecosystem impacts to understand differential impacts on eco-types and species of DWH and other hypothetical spills,
(d) Evaluation of the attributes of our selected V-R axes to confirm or refute their utility in predicting vulnerability and resilience to perturbation (and particularly to oil spills),
(e) Identification of ecosystem indicators sensitive to different environmental conditions,
(f) Understanding the natural variability of populations/assemblages within the GoM and over differing spatial scales,
(g) Understanding the population structure and connectivity of GoM biota and the relationship of genetic population structure to vulnerability and resilience.
These associated research recommendations address our contention (noted in the introduction) that, rather than four isolated eco-types, there are strong links among them due to physical oceanographic processes (advection) and biotic dispersal mechanisms of various species (either active movement or passive drifting) and the criticality of various scales of spatio-temporal variability. Coupling ecological niche and biophysical models for species moving among the eco-types is essential for quantifying the rates of coupling among ecosystem components. Dynamic coupling of these mechanisms with transport models (e.g., Vaz et al., 2021) will allow the construction of more advanced predictive modeling of oil spill dynamics and effects and a deeper understanding of some mechanisms that promote resiliency of the GoM ecosystem as a whole. Transport models, especially those that couple biology and physics, can also be employed to examine population connectivity in holoplankton or meroplanktonic species (e.g., Paris et al., 2020). Advances in genetic sequencing and genomic and bioinformatic tools (e.g., Dubansky et al., 2013), including the rapid development of practical applications of e-DNA approaches, however, likely provide the most robust set of tools to examine population structure and connectivity. Moreover, advances in epigenetic ageing (Mayne et al., 2022; Weber et al., 2022) and genetic close-kin mark-recapture analysis (Marcy-Quay et al., 2020) means that DNA from tissue samples can be utilized to estimate age composition, genetic structure, and size of populations. Further developing these analytical approaches, especially for vulnerable, long-lived, or relatively rare taxa would certainly fit with the research as restoration theme proposed by Mengerink et al. (2014).
(3)Conducting research on cumulative effects of environmental co-stressors including chronic low-level contamination in relation to acute effects from large oil spills.
The GoM region is subject to a host of significant, ongoing and aperiodic stressors affecting the region’s wildlife, including intensive fishing, hypoxia, nutrient over-enrichment, ongoing discharges of produced waters (e.g., Lee and Neff, 2011; Murawski, 2020; National Academies of Sciences, Engineering and Medicine, 2022a), oil spills and other toxic contaminants, harmful algal blooms, invasive species, effects of extreme weather events, sea level rise and land subsidence, and a host of others (Figure 3). As we have explained, our vulnerability-resilience plots implicitly incorporate cumulative effects of multiple stressors as they relate to resource recovery because the observed trends in population trajectories are used in defining the resilience metric. It is likely that many of the region’s resources will not recover to pre-spill levels of abundance and certainly not in a symmetrical way to their resource depletion trajectories, in spite of both ongoing habitat and “compensatory” restoration activities. This is because the cumulative impacts of the spill and multiple other stressors act in additive and multiplicative ways to affect resources, as noted by Duarte et al. (2009). The science needs to progress from descriptive assessments of co-stressors to predictive models of how manipulation of some of them (those under proximal human control) can affect resource recovery rates and levels; addressing research recommendations noted above are pre-requisites to building such models.
Summary
The Deepwater Horizon incident resulted in (arguably) the most complex and diverse set of ecosystem impacts in the history of marine oil spills. Four major interconnected “eco-types” exhibited a wide array of impacts to species and assemblages of organisms, ranging from inconsequential to catastrophic. Even a decade plus after DWH, many affected species and taxonomic groups have not fully recovered and recovery times for some may range from decades to perhaps a century or more (e.g., Schwacke et al., 2017; Schwing et al., 2020).
The development of criteria for assessing of the vulnerability of resource populations to the DWH spill and corresponding resilience to its effects provides an important new context for understanding how sub-systems within the GoM large marine ecosystem may be impacted by future large-scale perturbations. Comparisons of V-R relationships among eco-types revealed that all systems exhibited a range of outcomes for key species.
At least two major chronic “reservoirs” of DWH oil/dispersant residues remain in the environment (deep benthic, coastal wetlands) with surprisingly little ongoing weathering of oil (i.e., still toxic) in some cases (e.g., Turner et al., 2019a). In the case of the deep offshore benthic oil reservoir, it may take 50-100 years before DWH oil is sufficiently buried to be unavailable to invertebrate and vertebrate bioturbators (Montagna and Girard, 2020; Schwing et al., 2020), although by some metrics a degree of functional diversity had returned to affected areas by 2014 (Guarinello et al., 2022). The deep ocean communities in the pelagic (Romero et al., 2018) and benthic realms (Schwing et al., 2020) are highly diverse biomes that are particularly vulnerable to oil spills and possibly to mitigation approaches such as the use of sub-surface dispersant injection. With high vulnerability and low resilience of many species to oil spills, the dearth of research and understanding of the consequences of spills particularly in the deep GoM is astonishing, particularly with the rapid and accelerating progression of the industry into this realm (Murawski et al., 2020). Previous renditions of Comparative Risk Analyses supporting the use of specific oil spill countermeasures have inadequately characterized the deep ocean in terms of its importance in contributing to biodiversity and critical ecosystem services in the GoM (e.g., French-McCay et al., 2018). Diel vertical migration is a daily “elevator” from the deep sea to the surface waters of the GoM, so sequestration of oil in mid-depths (even if it is indeed possible through the use of SSDI) may result in unintended consequences which include surfacing of contaminated animals and food chain impacts. There are historic data deficiencies evident among the deep-sea groups, which are inconsistent with their high ecological and economic value. Taxa perceived as commercially valuable based on extractive resources usage (i.e., fished stocks) are usually better studied, but this perception ignores the exceptionally high value of non-extractive goods and services (e.g., carbon sequestration through the carbon pump, the importance of biodiversity in maintaining ecosystem function, food web relationships). Given the magnitude of the deep ocean habitats in the GoM, these data gaps urgently need to be addressed and the roles of deep-sea species properly evaluated.
The continental shelf resources of the northern GoM are highly productive and support important human uses including commercial and recreational fisheries. Species along the shelf exhibited a variety of acute and chronic impacts from the spill (e.g., some recruitment reductions) which will have intermediate population impacts. The overall assessment was that the continental shelf resources were among the most resilient of the four eco-types, due to a variety of factors including the degree of population connectivity among resource segments (Patterson et al., 2023). However, other confounding issues such as the rapid increases in abundance of invasive lionfishes may have resulted in regime shifts in community structure and changes in continental shelf productivity (e.g., Lewis et al., 2020). It is unclear what role DWH may have played (if any) in exacerbating this shift or depressing recovery of some species.
Nearshore resources, in particular, were significantly impacted by a combination of the oil spill and various spill countermeasures that were deployed, some of which may have had more consequential negative impacts on species than the oil contamination itself. Nearshore countermeasures including the construction of sand berms and freshwater releases were not only arguably ineffective at stemming oil contamination, but in some instances counterproductive to protection of populations, species and ecosystems.
Countermeasures deployed offshore in deep waters and on the continental shelf include in situ burning, extensive skimming at the surface and deployment of dispersants at the sea surface and sub-surface. A total of 411 in situ burns of surface oil were conducted during the DWH response, consuming 220-310 thousand barrels (bbl) of Macondo crude (Stout and Payne, 2016). Burn residues were relatively depleted of low molecular weight PAHs but enriched in pyrogenic PAHs including benzo[a]pyrene (Stout and Payne, 2016) a known carcinogen. The fate and impacts of DWH burn residues on living resources is largely unknown. Dispersant impacts are discussed extensively elsewhere (e.g., National Academies of Sciences, Engineering, and Medicine, 2020; National Academies of Sciences, Engineering and Medicine, 2022a).
While large-scale fishery closures were primarily intended to assure that tainted seafood did not reach consumers (Ylitalo et al., 2012), there were also some indications of short-term increases in resource abundance (e.g., shrimp and some finfishes) likely due to the closures, but these effects were ephemeral (Murawski et al., 2021a). The focus of fishery closures on surface expression of DWH oil did not fully account for low yet toxic concentrations for sub-surface transport of oil, which, to a certain degree, resulted in substantial oil flux and impacts on resources beyond fishery closure boundaries (Berenshtein et al., 2020).
There are concerns that DWH impacts may have weakened the resilience of resources via Allee effects (e.g., mate limitation, loss of genetic diversity, interrupting chemical signaling pathways, Schlenker et al., 2022), making them more susceptible to a range of other ongoing stressors (Greening et al., 2022). Long-term ecosystem remediation programs (e.g., marsh reconstruction through water diversions) may very well have consequential long-term negative impacts for some of ecologically and economically important resources initially affected by the DWH scenario (e.g., American oyster, bottlenose dolphin and a variety of finfish and shellfish of dependent on brackish habitats). The scale of restoration activities in the northern Gulf of Mexico, however, is so immense, it begs the question of their long term effectiveness in restoring species and ecosystems, and understanding which restoration activities will be most effective (National Academies of Sciences, Engineering, and Medicine, 2022b; Greening et al., 2022).
Author contributions
All authors contributed equally to the design, analysis, evaluation and publication writing leading to this contribution. All authors contributed to the article and approved the submitted version.
Funding
Funding for the project was primarily provided by the Gulf of Mexico Research Initiative through several of its research centers including: the Center for Integrated Modeling and Analysis of Gulf Ecosystems (C-IMAGE), Deep Pelagic Nekton Dynamics of the Gulf of Mexico (DEEPEND) and Ecosystem Impacts of Oil & Gas Inputs to the Gulf (ECOGIG).
Acknowledgments
In particular, we thank the Gulf of Mexico Research Initiative (GoMRI) Core Area 3 “motivators” (Rick Shaw, Margaret Leinen, Dick Dodge, Bill Hogarth, Bob Shipp, John Farrington, Ken Halanych, and John Shepherd) for their help and encouragement. As well, the staffs of the GoMRI Research Board and American Institute of Biological Sciences, who provided travel and logistical support, including Chuck Wilson, Michael Feldman, Callan Yanoff, and Jennifer Pettit. Liesl Hotaling assisted in coordinating the workshops we conducted leading to this publication and Diego Arenas developed options for Figure 2. We appreciate the work of our Research Topic editors, including Donald Boesch, Just Cebrian, Stephen Landers, Vanessa Fonseca, and Jay Rooker. For this paper we appreciate the insightful reviews of Edward Mager and Prosanta Chakrabarty and our review editor Donald Boesch.
Conflict of interest
The authors declare that the research was conducted in the absence of any commercial or financial relationships that could be construed as a potential conflict of interest.
Publisher’s note
All claims expressed in this article are solely those of the authors and do not necessarily represent those of their affiliated organizations, or those of the publisher, the editors and the reviewers. Any product that may be evaluated in this article, or claim that may be made by its manufacturer, is not guaranteed or endorsed by the publisher.
References
Adger W. N. (2006). Vulnerability. Glob. Env. Chan. 16, 268–281. doi: 10.1016/j.gloenvcha.2006.02.006
Adger W. N., Hughes T. P., Folke C., Carpenter S. R., Rockstrom J. (2005). Social-ecological resilience to coastal disasters. Science 309, 1036–1039. doi: 10.1126/science.1112122
Ainsworth C. H., Paris C. B., Perlin N., Dornberger L. N., Patterson W. F. III, Chancellor E., et al. (2018). Impacts of the Deepwater horizon oil spill evaluated using an end-to-end ecosystem model. PloS One 13, 1–21. doi: 10.1371/journal.pone.0190840
Anjos L. J. S., de Toledo P. M. (2018). Measuring resilience and assessing vulnerability of terrestrial ecosystems to climate change in south America. PloS One 13, e0194654. doi: 10.1371/journal.pone.0194654
Barron M. G., Vivian D. N., Heintz R. A., Hyuk U. (2020). Long-term ecological impacts from oil spills: comparison of Exxon valdez, Hebei spirit, and Deepwater horizon. Environ. Sci. Technol. 54 (11), 6456–6467. doi: 10.1021/acs.est.9b05020
Berenshtein I., Paris C. B., Perlin N., Alloy M. M., Joye S. B., Murawski S. (2020). Invisible oil beyond the Deepwater horizon satellite footprint. Sci. Adv. 6, eaaw8863. doi: 10.1126/sciadv.aaw8863
Beyer J., Trannum H. C., Bakke T., Hodson P. V., Collier T. K. (2016). Environmental effects of the Deepwater horizon oil spill: a review. Mar. Poll. Bull. 110, 28–51. doi: 10.1016/j.marpolbul.2016.027
Bodkin J. L., Esler D., Rice S. D., Matkin C. O., Ballachey B. E. (2014). “The effects of spilled oil on coastal ecosystems: lessons from the Exxon Valdez spill,” in Coastal conservation. Eds. Maslo B., Lockwood J. L. (NY: Cambridge University Press), 311–346.
Boesch D. F. (2020). Envisioning the future of the Louisiana gulf coast (Bentonville, AR, USA: Walton Family Foundation), 86. Available at: https://www.waltonfamilyfoundation.org/learning/envisioning-the-future-of-the-louisiana-gulf-coast.
Buskey E. J., White H. K., Esbaugh A. J. (2016). Impact of oil spills on marine life in the gulf of Mexico: effects on plankton, nekton, and deep-sea benthos. Oceanogr 29, 174–181. doi: 10.5670/oceanog.2016.81
Camilli R., Reddy C. M., Yoerger D. R., Van Mooy B. A. S., Jakuba M. V., Kinsey J. C., et al. (2010). Tracking hydrocarbon plume transport and biodegradation at Deepwater horizon. Science 330, 201–204. doi: 10.1126/science.1195223
Carlson S. M., Satterthwatte W. H. (2011). Weakened portfolio effect in a collapsed salmon population complex. Can. J. Fish. Aquat. Sci. 68, 1579–1589. doi: 10.1139/f2011-084
Carpenter S., Walker B., Anderies J. M., Abel N. (2001). From metaphor to measurement: resilience of what to what? Ecosys 4, 765–781. doi: 10.1007/s10021-001-0045-9
Carter N. H., Viña A., Hull V., McConnell W. J., Axinn W., Ghimire D., et al. (2014). Coupled human and natural systems approach to wildlife research and conservation. Ecol. Soc 19, 43. doi: 10.5751/ES-06881-190343
Chakrabarty P., Lam C., Hardman J., Aaronson J., House P. H., Janies D. A. (2012). Species map: a web-based application for visualizing the overlap of distributions and pollution events, with a list of fishes put at risk by the 2010 gulf of Mexico oil spill. Biodiv. Cons. 21, 1865–1876. doi: 10.1007/s10531-012-0284-4
Chakrabarty P., O’Neill G. A., Hardy B., Ballengee B. (2016). Five years later: an update on the status of collections of endemic gulf of Mexico fishes put at risk by the 2010 oil spill. Biodiv. Data J. 4, 1–27. doi: 10.3897/BDJ.4.e8728
Chancellor E. (2022) Quantifying environmental sensitivity of marine resources to oil well blowouts in the gulf of Mexico. Ph.D. Dissertation. University of South Florida, St. Petersburg, FL USA. Available at: https://digitalcommons.usf.edu/etd/9755.
Chancellor E., Murawski S. A., Paris C. B., Perruso L., Perlin N. (2020). “Comparative environmental sensitivity of offshore gulf of Mexico waters potentially impacted by ultra-deep oil well blowouts,” in Scenarios and responses to future deep oil spills - fighting the next war. Eds. Murawski S. A., Hollander D., Ainsworth C., Gilbert S., Paris C. B., Schlüter M., Wetzel D. (Springer Nature), 443–466. doi: 10.1007/978-3-030-12963-7
Coastal Protection and Restoration Authority of Louisiana (2017). Louisiana’s comprehensive master plan for a sustainable coast. Available at: http://coastal.la.gov/wp-content/uploads/2017/04/2017-Coastal-Master-Plan_Web-Single-Page_CFinal-with-Effective-Date-06092017.pdf.
Coastal Protection and Restoration Authority of Louisiana (2023). Louisiana’s comprehensive master plan for a sustainable coast (Baton Rouge, LA: Coastal Protection and Restoration Authority of Louisiana), 101. Available at: https://coastal.la.gov/wp-content/uploads/2023/04/230412_CPRA_MPFinal-for-webspreads.pdf.
Cockrell M. L., O’Farrell S., Sanchirico J., Murawski S. A., Perruso L., Strelcheck A. (2019). Resilience of a commercial fishing fleet following emergency closures in the gulf of Mexico. Fish. Res. 218, 69–82. doi: 10.1016/j.fishres.2019.04.017
Daly K. L., Passow U., Chanton J., Hollander D. (2016). Assessing the impacts of oil-associated marine snow formation and sedimentation during and after the Deepwater horizon oil spill. Anthropoc 13, 18–33. doi: 10.1016/j.ancene.2016.01.006
Deepwater Horizon Natural Resource Damage Assessment Trustees (2016). Deepwater horizon oil spill: final programmatic damage assessment and restoration plan and final programmatic environmental impact statement. Available at: http://www.gulfspillrestoration.noaa.gov/restoration-planning/gulf-plan.
De Lange H. J., Lahr J., van der Pol J. J. C., Wessels Y., Faber J. H. (2010b). Ecological vulnerability in wildlife: an expert judgement and multicriteria analysis tool using ecological traits to assess relative impact of pollutants. Env. Tox. Chem. 28, 2233–2240. doi: 10.1897/08-626.1
Diercks A.-R., Highsmith, R. C., Asper, V. L., Joung, D., Zhou, Z., et al. (2010). Characterization of subsurface polycyclic aromatic hydrocarbons at the Deepwater Horizon site. Geophys. Res. Lett. 37, L20602. doi: 10.1029/2010GL045046
De Lange H. J., Sala S., Vighi M., Faber J. H. (2010a). Ecological vulnerability in risk assessment – a review and perspectives. Sci. Tot. Env. 408, 3871–3879. doi: 10.1015/j.scitotenv.2009.11.009
Dornberger L. N., Ainsworth C. H., Coleman F., Wetzel D. L. (2020). “A synthesis of top-down and bottom-up impacts of the deepwater horizon oil spill using ecosystem modeling. chapter 31,” in Deep oil spills: facts, fate and effects Springer nature Eds. Murawski S. A., Hollander D., Ainsworth C., Gilbert S., Paris C. B., Schlüter M., Wetzel D. (Springer Nature). doi: 10.1007/978-3-030-11605-7
Duarte C. M., Conley D. J., Carstensen J., Sánchez-Camacho M. (2009). Return to Neverland: shifting baselines affect eutrophication restoration targets. Est. Coast. 32, 29–36. doi: 10.1007/s12237-008-9111-2
Dubansky B., Whitehead A., Miller J. T., Rice C. D., Galvez F. (2013). Multitissue molecular, genomic, and developmental effects of the deepwater horizon oil spill on resident gulf killifish (Fundulus grandis). Env. Sci. Tech. 47, 5074–5082. doi: 10.1021/es400458p
Esler D., Ballachey B. E., Matkin C., Cushing D., Kaler R., Bodkin J., et al. (2018). Timelines and mechanisms of wildlife population recovery following the Exxon valdez oil spill. Deep-Sea Res. Pt. II 147, 36–42. doi: 10.1016/j.dsr2.2017.04.007
Ferraroa P. J., Sanchirico J. N., Smith M. D. (2019). Causal inference in coupled human and natural systems. Proc. Nat. Acad. U.S.A. 116, 5311–5318. doi: 10.1073/pnas.1805563115
Field J., Cope J., Key M. (2010). A descriptive example of applying vulnerability evaluation criteria to California nearshore finfish species 2010. Manag. Data-Poor Fisheries: Case Studies Models Solutions 1, 235–246.
Fisher C. R., Montagna P. A., Sutton T. T. (2016). How did the Deepwater horizon oil spill impact deep-sea ecosystems? Oceanogr 29, 182–195. doi: 10.5670/oceanog.2016.82
Fodrie J., Able K. W., Galvez F., Heck K. L. Jr., Jensen O. P., Lopez-Duarte P. C., et al. (2014). Integrating organismal and population responses of estuarine fishes in macondo spill research. Biosci 64, 778–788. doi: 10.1093/biosci/biu123
Fraser K. E. (2020). “Evaluating impacts of deep oil spills on oceanic marine mammals. chapter 25,” in Scenarios and responses to future deep oil spills - fighting the next war. Eds. Murawski S. A., Hollander D., Ainsworth C., Gilbert S., Paris C. B., Schlüter M., Wetzel D. (Cham, Switzerland: Springer Nature). doi: 10.1007/978-3-030-12963-7
French-McCay D., Crowley D., Rowe J. J., Bock M., Robinson H., Wenning R., et al. (2018). Comparative risk assessment of spill response options for a deepwater oil well blowout: part 1. oil spill modeling. Mar. Poll. Bull. 133, 1001–1015. doi: 10.1016/j.marpolbul.2018.05.042
Gallaway B. J., Gazey W. J., Wibbels T., Bevan E., Shaver D. J., George J. (2016). Evaluation of the status of the kemp’s ridley sea turtle after the 2010 Deepwater horizon oil spill. Gulf Mex. Sci. 33, 192–205. doi: 10.18785/goms.3302.06
Greening H., Heck K. L., McKinney L. D., Diefenderfer H. L., Boynton W. R., Kleiss B. A., et al. (2022). Estuar. Coasts 46, 293–301. doi: 10.1007/s12237-022-01149-8
Grilli J., Rogers T., Allesina S. (2016). Modularity and stability in ecological communities. Nat. Comm 7, 1–10. doi: 10.1038/ncomms12031
Gros J., Socolofsky S. A., Dissanayake A. L., Jun I., Zhao L., Boufadel M. C., et al. (2017). Petroleum dynamics in the sea and influence of subsea dispersant injection during deepwater horizon. Proc. Nat. Acad. Sci. 114, 10065–10070. doi: 10.1073/pnas.1612518114
Grosell M., Pasparakis C. (2021). Physiological responses of fish to oil spills. Ann. Rev. Mar. Sci. 13, 12.1–12.24. doi: 10.1146/annurev-marine-040120-094802
Guarinello M. L., Sturdivant, S. K., Murphy, A. E., Brown, L., Godbold, J. A., Solan, M., et al. (2022). Evidence of rapid functional benthic recovery following the Deepwater Horizon oil spill. ACS EST Water 2 (10), 1760–1771. doi: 10.1021/acsestwater.2c00272
Gunderson L. (2000). Ecological resilience in theory and application. Annu. Rev. Ecol. Syst. 31, 425–439. doi: 10.1146/annurev.ecolsys.31.1.425
Gunderson L. H., Prichard J. L. (2002). Resilience and the behavior of large-scale systems (Washington, DC: Island Press), 287.
Halanych K. H., Ainsworth C. H., Cordes E. E., Dodge R. E., Huettel M., Mendelssohn I. A., et al. (2021). Effects of petroleum by-products and dispersants on ecosystems. Oceanography 34, 152–163. doi: 10.5670/oceanog.2021.123
Hobday A. J., Smith A. D. M., Stobutzki I. C., Bulman C., Daley R., Dambacher J. M., et al. (2011). Ecological risk assessment for the effects of fishing. Fish. Res. 108, 372–384. doi: 10.1016/j.fishres.2011.01.013
Holling C. S. (1973). Resilience and stability of ecological systems. Annu. Rev. Ecol. Syst. 4, 1–23. doi: 10.1146/annurev.es.04.110173.000245
Hordyk A. R., Carruthers T. R. (2018). A quantitative evaluation of a qualitative risk assessment framework: examining the assumptions and predictions of the productivity susceptibility analysis (PSA). PloS One 13, e0198298. doi: 10.1371/journal.pone.0198298
Joye S. B., MacDonald I. R., Leifer I., Asper V. (2011). Magnitude and oxidation potential of hydrocarbon gases released from the BP blowout. Nat. Geosci. 4, 160–164. doi: 10.1038/ngeo1067
Kostka J. E., Prakash O., Overholt W. A., Green S. J., Freyer G., Canion A., et al. (2011). Hydrocarbon-degrading bacteria and the bacterial community response in gulf of Mexico beach sands impacted by the Deepwater horizon oil spill. Appl. Env. Microb. 77 (7), 962–967. doi: 10.1128/AEM.05402-11
Lee K.,, Neff J. (Eds). (2011). Produced water: environmental risks and advances in mitigation technologies. New York: Springer Science + Business Media. doi: 10.1007/978-1-4614-2
Levin S. A., Lubchenco J. (2008). Resilience, robustness, and marine ecosystem-based management. BioSci 8, 27–32. doi: 10.1641/B580107
Lewis J. P., Tarnecki J. H., Garner S. B., Chagaris D. D., Patterson III, W. F. (2020). Changes in reef fish community structure following the Deepwater horizon oil spill. Sci. Reps. 10, 5621. doi: 10.1038/s41598-020-62574-y
Lubchenco J., McNutt M. K., Dreyfus G., Murawski S. A., Kennedy D. M., Anastas P. T., et al. (2012). Science in support of the Deepwater horizon response. Proc. Nat. Acad. Sci. U.S.A. 109, 20212–20221. doi: 10.1073/pnas.1204729109
MacDonald I. R., Garcia-Pineda O., Beet A., Daneshgar Asl S., Feng L., Graettinger G., et al. (2015). Natural and unnatural oil slicks in the gulf of Mexico. J. Geophys. Res. Ocean. 120, 8364–8380. doi: 10.1002/2015JC011062
Marcy-Quay B., Sethi S. A., Therkildsen N. O., Kraft C. E. (2020). Expanding the feasibility of fish and wildlife assessments with close-kin mark–recapture. Ecosphere 11, e03259. doi: 10.1002/ecs2.3259
Martínez M. L., Feagin R. A., Yeager K. M., Day J., Costanza R., Harris J. A., et al. (2012). Artificial modifications of the coast in response to the Deepwater horizon oil spill: quick solutions or longterm liabilities? Front. Ecol. Environ. 10, 44–49. doi: 10.1890/100151
Mayne B., Mustin W., Baboolal V., Casella F., Ballorain K., Barret M., et al. (2022). Age prediction in green turtles with an epigenetic clock. Mol. Ecol. Resour. 22, 2275–2284. doi: 10.1111/1755-0998.13621
McCann M. J., Able K. W., Christian R. R., Fodrie F. J., Jensen O., Johnson J. J., et al. (2017). Key taxa in food web responses to stressors: the Deepwater horizon oil spill. Front. Ecol. Environ. 15, 142–149. doi: 10.1002/fee.1474
McNutt M. K., Chu S., Lubchenco J., Hunter T., Dreyfus G., Murawski S. A., et al. (2012). Applications of science and engineering to quantify and control the Deepwater horizon oil spill. Proc. Nat. Acad. Sci. U.S.A. 109, 20222–20228. doi: 10.1073/pnas.1214389109
Mengerink K., Van Dover C. L., Ardron J., Baker M., Escobar-Briones E., Gjerde K., et al. (2014). A call for deep-ocean stewardship. Science 344, 696–698. doi: 10.1126/science.1251458
Michael P. E., Hixson K. M., Haney J. C., Satgé Y. G., Gleason J. S., Jodice P. G. R. (2022). Seabird vulnerability to oil: exposure potential, sensitivity, and uncertainty in the northern gulf of Mexico. Front. Mar. Sci. 9. doi: 10.3389/fmars.2022.880750
Milligan R. J., Sutton T. T. (2020). Dispersion overrides environmental variability as a primary driver of the horizontal assemblage structure of the mesopelagic fish family myctophidae in the northern gulf of Mexico. Front. Mar. Sci. 7. doi: 10.3389/fmars.2020.00015
Montagna P. A., Girard F. (2020). “Deep-sea benthic faunal impacts and community evolution before, during and after the deepwater horizon event. chapter 22,” in Deep oil spills: facts, fate and effects. Eds. Murawski S. A., Hollander D., Ainsworth C., Gilbert S., Paris C. B., Schlüter M., Wetzel D. (Cham, Switzerland: Springer Nature). doi: 10.1007/978-3-030-11605-7
Murawski S. A. (2020). “Perspectives on research, technology, policy and human resources for improvement management of ultra-deep oil and gas resources and responses to oil spills. chapter 29,” in Scenarios and responses to future deep oil spills - fighting the next war. Eds. Murawski S. A., Hollander D., Ainsworth C., Gilbert S., Paris C. B., Schlüter M., Wetzel D. (Cham, Switzerland: Springer Nature). doi: 10.1007/978-3-030-12963-7
Murawski S. A., Fleeger J. W., Patterson W. F. III, Hu C., Daly K., Romero I., et al. (2016). How did the Deepwater horizon oil spill affect coastal and continental shelf ecosystems of the gulf of Mexico? Oceanogr 29, 160–173. doi: 10.5670/oceanog.2016.80
Murawski S. A., Grosell M., Smith C., Sutton T., Halanych K. M., Shaw R. F., et al. (2021b). Impacts of petroleum, petroleum components, and dispersants on organisms and populations. Oceanography 34, 136–151. doi: 10.5670/oceanog.2021.122
Murawski S. A., Hogarth W. T., Peebles E. B., Barbieri L. (2014). Prevalence of external skin lesions and polycyclic aromatic hydrocarbon concentrations in gulf of Mexico fishes, post-Deepwater horizon. Trans. Am. Fish. Soc 143, 1084–1097. doi: 10.1080/00028487.2014.911205
Murawski S. A., Hollander D., Gilbert S., Gracia A. (2020). “Deep-water oil and gas production in the gulf of Mexico, and related global trends. chapter 2,” in Scenarios and responses to future deep oil spills - fighting the next war. Eds. Murawski S. A., Hollander D., Ainsworth C., Gilbert S., Paris C. B., Schlüter M., Wetzel D. (Cham, Switzerland: Springer Nature). doi: 10.1007/978-3-030-12963-7
Murawski S. A., Kilborn J. P., Bejarano A., Chagaris D., Donaldson D., Hernandez J. F.J., et al. (2021a). A synthesis of impacts of Deepwater horizon on coastal and nearshore living marine resources. Front. Mar. Sci. 7. doi: 10.3389/fmars.2020.594862
Murawski S. A., Schlüter M., Paris C. B., Aman Z. M. (2019). Resolving the dilemma of dispersant use for deep oil spill response. Environ. Res. Lett. 14, 091002. doi: 10.1088/1748-9326/ab3aa0
National Academies of Sciences, Engineering, and Medicine (2020). The use of dispersants in marine oil spill response (Washington, DC: The National Academies Press). doi: 10.17226/25161
National Academies of Sciences, Engineering and Medicine (2022a). Oil in the Sea IV: inputs, fates and effects (Washington DC: The National Academies Press). doi: 10.17226/26410
National Academies of Sciences, Engineering, and Medicine (2022b). An approach for assessing U.S. gulf coast ecosystem restoration: a gulf research program environmental monitoring report (Washington, DC: The National Academies Press). doi: 10.17226/26335
O’Connell M. T., Peterson M. S., Powers S. P., Uzee-O’Connell A. M., Anderson E. J., Hendon J. R. (2019). Assessing nearshore nekton abundance, substrate, and environmental conditions in the northern gulf of Mexico: are there differences among three adjacent coastal areas and have there been changes over three decades? Est. Coasts. 42, 2139–2169 doi: 10.1007/s12237-019-00632-z
Paris C. B., Berenshtein I., Trillo M. L., Faillettaz R., Olascoaga M. J., Aman Z., et al. (2018). BP Gulf science data reveals ineffectual sub-sea dispersant injection for the macondo blowout. Front. Mar. Sci. doi: 10.3389/fmars.2018.00389
Paris C. B., Le Hénaff M., Aman Z. M., Subramanian A., Helgers J., Wang D.-P., et al. (2012). Evolution of the macondo well blowout: simulating the effects of the circulation and synthetic dispersants on the subsea oil transport. Env. Sci. Tech. 46, 13293–13302. doi: 10.1021/es303197h
Paris C. B., Murawski S. A., Olascoaga M. J., Vaz A. C., Berenshtein I., Miron P., et al. (2020). “Connectivity of the gulf of Mexico continental shelf fish populations and implications of simulated oil spills. chapter 22,” in Scenarios and responses to future deep oil spills - fighting the next war. Eds. Murawski S. A., Hollander D., Ainsworth C., Gilbert S., Paris C. B., Schlüter M., Wetzel D. (Cham, Switzerland: Springer Nature). 5:389, 1–9. doi: 10.1007/978-3-030-12963-7
Patrick W. S., Spencer P., Link J., Cope J., Field J., Kobayashi D., et al. (2010). Using productivity and susceptibility indices to assess the vulnerability of united states fish stocks to overfishing. Fish Bull.(U.S.) 108, 305–322.
Patterson W. F. III, Robinson K. L., Barnett B. K., Campbell M., Chagaris D. C., Daly K., et al. (2023). Population-level impacts and resiliency for Gulf of Mexico shelf taxa following the Deepwater horizon oil spill. Front. Mar. Sci.
Peterson C. H., Anderson S., Cherr G., Ambrose R., Anghera S., Bay S., et al. (2012). A tale of two spills. BioScience 62, 461–469. doi: 10.1525/bio.2012.62.5.7
Peterson C. H., Rice S. D., Short J. W., Esler D., Bodkin J. L., Ballachey B. E., et al. (2003). Long-term ecosystem response to the Exxon valdez oil spill. Science 302, 2082–2086. doi: 10.1126/science.1084282
Polidoro B., Matson C. W., Ottinger M. A., Renegar D. A., Romero I. C., Schlenk D., et al. (2020). A multi-taxonomic framework for assessing relative petrochemical vulnerability of marine biodiversity in the gulf of Mexico. Sci. Tot. Environ. 763, 142986. doi: 10.1016/j.scitotenv.2020.142986
Powers S., Grabowski J. H., Roman H., Geggel A., Rouhani S., Oehrig J., et al. (2017b). Consequences of large-scale salinity alteration during the Deepwater horizon oil spill on subtidal oyster populations. Mar. Ecol. Prog. Ser. 576, 175–187. doi: 10.3354/meps12147
Powers S. P., Peterson C. H., Cebrian J., Heck J. K.L. (2017a). Response of nearshore ecosystems to the deepwater horizon spill. Mar. Ecol. Prog. Ser. 576, 107–110. doi: 10.3354/meps12254
Rabalais N. N., Turner R. E. (2016). Effects of the Deepwater horizon oil spill on coastal marshes and associated organisms. Oceanogr 29, 150–159. doi: 10.5670/oceanog.2016.79
Ramírez-León M. R., Sosa-Nishizaki O., Pérez-Brunius P., Romo-Curiel A. E., Ramíırez-Mendoza Z., Fajardo-Yamamoto A., et al. (2023). Semi-quantitative risk assessment of marine mammal oil exposure: a case study in the western gulf of Mexico. Front. Mar. Sci. 10:1034647, 1-14. doi: 10.3389/fmars.2023.1034647
Romero I. C., Gerardo Toro-Farmer G., Diercks A.-R., Schwing P., Muller-Karger F., Murawski S., et al. (2017). Large-Scale deposition of weathered oil in the gulf of Mexico following a deep-water oil spill. Env. Poll. 228, 179–189. doi: 10.1016/j.envpol.2017.05.0190269-749
Romero I. C., Sutton T., Carr B., Quintana-Rizzo E., Ross S. W., Hollander D. J., et al. (2018). Decadal assessment of polycyclic aromatic hydrocarbons in mesopelagic fishes from the gulf of Mexico reveals exposure to oil-derived sources. Environ. Sci. Technol. 52, 10985–10996. doi: 10.1021/acs.est.8b02243
Rowe G. T., Kennicutt M. C. (2008). Introduction to the deep gulf of Mexico benthos program. Deep-Sea Res. II 55, 2536–2540. doi: 10.1016/j.dsr2.2008.09.002
Ryerson T. B., Camilli R., Kessler J. D., Kujawinski E. B., Reddy C. M., Valentine D. L., et al. (2012). Chemical data quantify Deepwater horizon hydrocarbon flow rate and environmental distribution. Proc. Nat. Acad. Sci. U.S.A. 109, 20246–20253. doi: 10.1073/pnas.1110564109
Schindler D. E., Hilborn R., Chasco B., Boatright C. P., Quinn T. P., Rogers L. A., et al. (2010). Population diversity and the portfolio effect in an exploited species. Nature 465, 609–612. doi: 10.1038/nature09060
Schlenker L. S., Stieglitz J. D., Greer J. B., Faillettaz R., Lam C. H., Hoenig R. H., et al. (2022). Brief oil exposure reduces fitness in wild gulf of Mexico mahi-mahi (Coryphaena hippurus). Environ. Sci. Technol. 56 (18), 13019–13028. doi: 10.1021/acs.est.2c01783
Schwacke L. H., Smith C. R., Townsend F. I., Wells R. S., Hart L. B., Balmer B. C., et al. (2014). Health of common bottlenose dolphins (Tursiops truncatus) in barataria bay, Louisiana, following the Deepwater horizon oil spill. Environ. Sci. Technol. 48, 93–103. doi: 10.1021/es403610f
Schwacke L. H., Thomas L., Wells R. S., Mcfee W. E., Hohn A. A., Mullin K. D., et al. (2017). Quantifying injury to common bottlenose dolphins from the Deepwater horizon oil spill using an age-, sex-and class-structured population model. Endang Species Res. 33, 265–279. doi: 10.3354/esr00777
Schwing P. T., Montagna P. A., Joye S., Paris C. B., Cordes E. E., McClain C. R., et al. (2020). Deep benthic ecosystem impacts of the Deepwater horizon event: assembling the record of species and community change. Front. Mar. Sci. 7. doi: 10.3389/fmars.2020.56001
Soto L. A., Botello A. V., Licea-Durán S., Lizárraga-Partida M. L., Yáñez-Arancibia A. (2014). The environmental legacy of the ixtoc-I oil spill in campeche sound, southwestern gulf of Mexico. Front. Mar. Sci. 1. doi: 10.3389/fmars.2014.00057
Stobutzki I., Miller M., Brewer D. (2001). Sustainability of fishery bycatch: a process for assessing highly diverse and numerous bycatch. Environ. Conserv. 28, 167–181. doi: 10.1017/S0376892901000170
Stout S. A., Payne J. R. (2016). Chemical composition of floating and sunken in-situ burn residues from the deepwater horizon oil spill. Mar. Poll. Bull. 108, 186–202. doi: 10.1016/j.marpolbul.2016.04.031
Sutton T. T., Frank T., Judkins H., Romero. I. C. (2020). “As gulf oil extraction goes deeper, who is at risk? community structure, distribution, and connectivity of the deep-pelagic fauna, chapter 24,” in Scenarios and responses to future deep oil spills - fighting the next war. Eds. Murawski S. A., Hollander D., Ainsworth C., Gilbert S., Paris C. B., Schlüter M., Wetzel D. (Cham, Switzerland: Springer Nature). doi: 10.1007/978-3-030-12963-7
Sutton T. T., Milligan R. J., Daly K., Boswell K. M., Cook A. B., Cornic M., et al. (2022). The open-ocean gulf of Mexico after deepwater horizon: synthesis of a decade of research. Front. Mar. Sci. 9. doi: 10.3389/fmars.2022.753391
Turner R. E., Layne M., Mo Y., Swensen E. M. (2019b). Net land gain or loss for two Mississippi river diversions: caernarvon and Davis pond. Restor. Ecol. 27 (6), 1231-1240. doi: 10.1111/rec.13024
Turner R. E., Rabalais N. N., Overton E. B., Meyer B. M., McClenachan G., Swenson E. M., et al. (2019a). Oiling of the continental shelf and coastal marshes over eight years after the 2010 Deepwater horizon oil spill. Environ. Poll. 252, 1367–1376. doi: 10.1016/j.envpol.2019.05.134
Vaz A. C., Faillettaz R., Paris C. B. (2021). A coupled Lagrangian-earth system model for predicting oil photooxidation. Front. Mar. Sci. 8. doi: 10.3389/fmars.2021.576747
Wallace R. L., Gilbert S., Reynolds J. E. III (2019). Improving the integration of restoration and conservation in marine and coastal ecosystems: lessons from the Deepwater horizon disaster. BioSci 69, 920–927. doi: 10.1093/biosci/biz103
Weber D. N., Fields A. T., Patterson W. F. III, Barnett B. K., Hollenbeck C. M., Portnoy D. S. (2022). Novel epigenetic age estimation in wild-caught gulf of Mexico reef fishes. Can. J. Fish. Aquat. Res. 79, 1–5. doi: 10.1139/cjfas-2021-0240
Weißhuhn P., Müller F., Wiggering H. (2018). Ecosystem vulnerability review: proposal of an interdisciplinary ecosystem assessment approach. Environ. Manage. 61, 04–915. doi: 10.1007/s00267-018-1023-8
White J. R., Couvillion B., Day J. W. (2023). Coastal wetland area change for two freshwater diversions in the Mississippi river delta. Ecol. Engin 186, 106819. doi: 10.1016/j.ecoleng.2022.106819
White E. D., Messina F., Moss L., Meselhe E. (2018). Salinity and marine mammal dynamics in barataria basin: historic patterns and modeled diversion scenarios. Water 10, 1015. doi: 10.3390/w10081015
Woodyard M., Polidoro B. A., Matson C. W., McManamay R. A., Saul S., Carpenter K. E., et al. (2022). A comprehensive petrochemical vulnerability index for marine fishes in the gulf of Mexico. Sci. Tot. Environ. 820, 152892. doi: 10.1016/j.scitotenv.2021.152892
Keywords: ecosystem vulnerability, ecosystem resilience, marine oil spills, Deepwater Horizon, Gulf of Mexico
Citation: Murawski SA, Schwing PT, Patterson WF III, Sutton TT, Montagna PA, Milligan RJ, Joye SB, Thomas L, Kilborn JP, Paris CB, Faillettaz R, Portnoy DS and Gilbert S (2023) Vulnerability and resilience of living marine resources to the Deepwater Horizon oil spill: an overview. Front. Mar. Sci. 10:1202250. doi: 10.3389/fmars.2023.1202250
Received: 07 April 2023; Accepted: 30 May 2023;
Published: 14 June 2023.
Edited by:
Donald F. Boesch, University of Maryland, College Park, United StatesReviewed by:
Edward Mager, University of North Texas, United StatesProsanta Chakrabarty, Louisiana State University, United States
Copyright © 2023 Murawski, Schwing, Patterson, Sutton, Montagna, Milligan, Joye, Thomas, Kilborn, Paris, Faillettaz, Portnoy and Gilbert. This is an open-access article distributed under the terms of the Creative Commons Attribution License (CC BY). The use, distribution or reproduction in other forums is permitted, provided the original author(s) and the copyright owner(s) are credited and that the original publication in this journal is cited, in accordance with accepted academic practice. No use, distribution or reproduction is permitted which does not comply with these terms.
*Correspondence: Steven A. Murawski, smurawski@usf.edu